Osmotic Dehydration of Garden Egg (Solanum aethiopicum): the Shrinkage and Mass Transfer Phenomena
Article Information
Adekeye DK1*, Aremu OI1, Popoola OK1, Fadunmade EO1, Adedotun IS2, Araromi AA3
1Department of Chemistry, Ekiti State University Ado-Ekiti, Ekiti State
2Department of Pure and Applied Chemistry, Osun State University Osogbo, Osun State
3Department of Industrial Chemistry, Ekiti State University Ado-Ekiti, Ekiti State
*Corresponding Author: Adekeye DK, Department of Chemistry, Ekiti State University Ado-Ekiti, Ekiti State
Received: 26 November 2019; Accepted: 09 January 2020; Published: 11 January 2020
Citation: Adekeye DK, Aremu OI, Popoola OK, Fadunmade EO, Adedotun IS, Araromi AA. Osmotic Dehydration of Garden Egg (Solanum aethiopicum): the Shrinkage and Mass Transfer Phenomena. Journal of Analytical Techniques and Research 2(2020): 1-17.
Share at FacebookAbstract
Osmotic dehydration is usually employed to lower the water activity of fruits and vegetables and thus has a wide range of application in the food preservation. This study investigates the osmotic dehydration of garden egg (Solanum aethiopicum) using sodium chloride solution (NaCl) of varying concentration (20, 40 and 60 %w/w) as osmotic solutions. Different parameters such as percentage water loss (%WL), weight reduction (%WR) and solid gain (%SG) were investigated at different solution temperature (25, 35, 45, 55, and 65°C), agitation time (20, 40, 60, 80, 100, 120, 140, 160 and 180 min) and osmotic solution concentration (20, 40 and 60 %w/w) to ascertain the optimum dehydration performance of the process. The performance efficiency of the process was evaluated at different osmotic solution concentration (20, 40 and 60 %w/w) with respect to time (20-180 min) at constant temperature (40 OC). The performance efficiency of the process was also evaluated at different osmotic solution concentration (20, 40 and 60 %w/w) with respect to temperature (25, 35, 45, 55, and 65°C) at constant time (180 min). The results of this study showed that %SG and %WL increased with increase in concentration with respect to time whereby the optimum values for these parameters being 157% and 45.80% respectively were achieved at 60 %w/w osmotic solution concentration, while optimum %WR (34.70%) with respect to time was achieved at J Anal Tech Res 2020; 3 (1): 1-17 DOI: 10.26502/jatri.009 Journal of Analytical Techniques and Research 2 40 %ww osmotic solution concentration. Therefore, the order of %SG and %WL by the garden egg with respect to time was 60 > 40 > 20 %w/w. The optimum %SG, %WL and %WR were achieved at 65 oC in 60 %w/w osmotic solution with the values of 133, 53.11 and 40.10% respectively. The results from this study therefore showed that osmotic dehydration could potentially reduce water activity of garden egg.
Keywords
Osmotic dehydration; Food preservation; Water activity; Garden egg; Rural area.
Osmotic dehydration articles, Food preservation articles, Water activity articles, Garden egg articles, Rural area articles
Osmotic dehydration articles Osmotic dehydration Research articles Osmotic dehydration review articles Osmotic dehydration PubMed articles Osmotic dehydration PubMed Central articles Osmotic dehydration 2023 articles Osmotic dehydration 2024 articles Osmotic dehydration Scopus articles Osmotic dehydration impact factor journals Osmotic dehydration Scopus journals Osmotic dehydration PubMed journals Osmotic dehydration medical journals Osmotic dehydration free journals Osmotic dehydration best journals Osmotic dehydration top journals Osmotic dehydration free medical journals Osmotic dehydration famous journals Osmotic dehydration Google Scholar indexed journals Food preservation articles Food preservation Research articles Food preservation review articles Food preservation PubMed articles Food preservation PubMed Central articles Food preservation 2023 articles Food preservation 2024 articles Food preservation Scopus articles Food preservation impact factor journals Food preservation Scopus journals Food preservation PubMed journals Food preservation medical journals Food preservation free journals Food preservation best journals Food preservation top journals Food preservation free medical journals Food preservation famous journals Food preservation Google Scholar indexed journals Water activity articles Water activity Research articles Water activity review articles Water activity PubMed articles Water activity PubMed Central articles Water activity 2023 articles Water activity 2024 articles Water activity Scopus articles Water activity impact factor journals Water activity Scopus journals Water activity PubMed journals Water activity medical journals Water activity free journals Water activity best journals Water activity top journals Water activity free medical journals Water activity famous journals Water activity Google Scholar indexed journals Garden egg articles Garden egg Research articles Garden egg review articles Garden egg PubMed articles Garden egg PubMed Central articles Garden egg 2023 articles Garden egg 2024 articles Garden egg Scopus articles Garden egg impact factor journals Garden egg Scopus journals Garden egg PubMed journals Garden egg medical journals Garden egg free journals Garden egg best journals Garden egg top journals Garden egg free medical journals Garden egg famous journals Garden egg Google Scholar indexed journals Rural area articles Rural area Research articles Rural area review articles Rural area PubMed articles Rural area PubMed Central articles Rural area 2023 articles Rural area 2024 articles Rural area Scopus articles Rural area impact factor journals Rural area Scopus journals Rural area PubMed journals Rural area medical journals Rural area free journals Rural area best journals Rural area top journals Rural area free medical journals Rural area famous journals Rural area Google Scholar indexed journals application in the food preservation articles application in the food preservation Research articles application in the food preservation review articles application in the food preservation PubMed articles application in the food preservation PubMed Central articles application in the food preservation 2023 articles application in the food preservation 2024 articles application in the food preservation Scopus articles application in the food preservation impact factor journals application in the food preservation Scopus journals application in the food preservation PubMed journals application in the food preservation medical journals application in the food preservation free journals application in the food preservation best journals application in the food preservation top journals application in the food preservation free medical journals application in the food preservation famous journals application in the food preservation Google Scholar indexed journals parameters articles parameters Research articles parameters review articles parameters PubMed articles parameters PubMed Central articles parameters 2023 articles parameters 2024 articles parameters Scopus articles parameters impact factor journals parameters Scopus journals parameters PubMed journals parameters medical journals parameters free journals parameters best journals parameters top journals parameters free medical journals parameters famous journals parameters Google Scholar indexed journals osmotic solution articles osmotic solution Research articles osmotic solution review articles osmotic solution PubMed articles osmotic solution PubMed Central articles osmotic solution 2023 articles osmotic solution 2024 articles osmotic solution Scopus articles osmotic solution impact factor journals osmotic solution Scopus journals osmotic solution PubMed journals osmotic solution medical journals osmotic solution free journals osmotic solution best journals osmotic solution top journals osmotic solution free medical journals osmotic solution famous journals osmotic solution Google Scholar indexed journals Analytical Techniques articles Analytical Techniques Research articles Analytical Techniques review articles Analytical Techniques PubMed articles Analytical Techniques PubMed Central articles Analytical Techniques 2023 articles Analytical Techniques 2024 articles Analytical Techniques Scopus articles Analytical Techniques impact factor journals Analytical Techniques Scopus journals Analytical Techniques PubMed journals Analytical Techniques medical journals Analytical Techniques free journals Analytical Techniques best journals Analytical Techniques top journals Analytical Techniques free medical journals Analytical Techniques famous journals Analytical Techniques Google Scholar indexed journals preservation technologies articles preservation technologies Research articles preservation technologies review articles preservation technologies PubMed articles preservation technologies PubMed Central articles preservation technologies 2023 articles preservation technologies 2024 articles preservation technologies Scopus articles preservation technologies impact factor journals preservation technologies Scopus journals preservation technologies PubMed journals preservation technologies medical journals preservation technologies free journals preservation technologies best journals preservation technologies top journals preservation technologies free medical journals preservation technologies famous journals preservation technologies Google Scholar indexed journals
Article Details
1. Introduction
It has been stated that about 20-40% percent of the fruit and vegetable production in many countries of the world goes waste due to lack of proper and adequate storage system [1]. Therefore, good storage system and preservation technologies are necessary to ensure the safety, extension of shelf life and the quality of foods. Several techniques have been developed to satisfy current demands of economic preservation of food and consumer satisfaction in safety, nutritional and health aspects. Some of these techniques include: heat treatment (pasteurisation and sterilisation) [2], Preservation by low water activity (aw) [3], Preservation by organic acids [4] Preservation by carbon dioxide [5] and Osmotic Dehydration [6, 7]. Osmotic dehydration aims at extending life of food by removing water without phase transition according to Matuska et al [8]. The process is carried out by immersing the raw material in a hypertonic solution. Optimization of osmotic process is done in order to achieve and ascertain optimum dehydration. This optimization includes optimization of: temperature, concentration, immersion time and mass of substance. When all this parameters are optimized, different variables such as mass loss, mass transfer gain, colour change etc, can be determined [9-11]. Osmotic dehydration has been known to be one of the best and suitable methods to increase the shelf life of fruits and vegetables. This process is preferred over others due to their vitamins and minerals, colour, flavour and taste retention property [1]. Several researchers have reported the potential of this process to reduce the perishability of fruits and vegetables by reducing their high water content [1, 12, 13]. For instance, Bchir et al. [14] reported the Osmotic dehydration of pomegranate seed carried out at different temperature (30, 40 and 50 °C) in 50 °Brix sucrose, glucose and mixture of two in 50:50. The results of their study showed 46% water loss in sucrose, 37% in glucose and 41% in mixture solution after 20 min; also, increase in temperature increases the water loss. Mercali et al. [15] also worked on optimization of osmotic dehydration of banana with respect to temperature (25 – 55 °C) salt (0 – 10 g/100 g) and sucrose (30 – 60 g/100 g) concentrations. The result of their study showed water loss and solid gain by the banana during the dehydration process. Several osmotic dehydration studies have been reported for various kinds of fruits and vegetables but none have been reported for garden egg (Solanum aethiopicum) to our best of knowledge.
Garden egg plants are fruit vegetables of some varieties which are white with egg-like shape, hence the name ‘eggplants’ [16]. Garden egg is cultivated all year round in different parts of Nigeria and West Africa and serves as one of the main source of income for many rural farmers and households. Eggplant nutritious value is comparable to the values of other common vegetables. Its fresh weight is composed of 1.4% protein, 93% moisture, 0.3% minerals, 1.3% fibre, 0.3% fat, and the remaining 4% consists of various carbohydrates and vitamins (A and C). It also contains water (about 92.5%), protein (1%), fat (0.3%), and carbohydrates (6%). Similarly, eggplant contains nutrients such as dietary fiber, vitamin K, niacin, vitamin B6, folate, ascorbic acid, magnesium, manganese, pantothenic acid, phosphorus, potassium, iron, and copper [17]. According to Okito et al [18], eggplant is most popular in southern Nigeria particularly in Igbo land, because of both cultural and traditional importance. In Nigeria, though there are no official figures recorded for Solanum gilo and Solanum aethiopicum production, the crop has a wide distribution as a garden crop [19]. Production of eggplant is however constrained by preservation technique, a wide range of pests and diseases reducing total production as well as production quality. This research is therefore aimed at investigating osmotic dehydration using salt solution as a possible preservation technique for garden egg.
2. Materials and Methods
2.1 Materials
Garden eggs (Solanum aethiopicum), salt (NaCl) solution of varying concentrations (20, 40 and 60 %w/w), analytical weighing balance, oven, stop watch, distilled water, volumetric cylinder, beakers, conical flasks, Aluminum foil and cotton wool.
2.2 Methods
2.2.1 Sample collection and preparation: The garden eggs which have no defects were sourced from a local market (Mojere) at Ado-Ekiti, Ekiti State, Nigeria. The plant was identified by the Herbarium curator at the Department of Plant Science and Biotechnology, Ekiti State University, Ado-Ekiti, Southwestern Nigeria. The samples were prepared by cleaning, washing with distilled water and air-drying at room temperature for 24 hours prior to the osmo-dehydration experiments. Thereafter, the samples were cut into slices (each slice weighing 10 g) enough to carry out the experiments.
2.2.2 Osmotic solution preparation: Osmotic solutions (50 mL) of varying concentration (20, 40 and 60% w/w) were prepared using analytical grade salt and distilled water by dissolving the appropriate amount of salt in 50 mL distilled.
2.3 Determination of physical parameters of the raw garden egg sample
2.3.1 Determination of density of the raw garden egg: The density of the garden egg sample was determined by estimating the weight of 10 g of the sample in 100 ml distilled water in a volumetric cylinder. The displaced volume was measured and density was calculated by Equation I. The experiment was conducted in triplicate.
2.3.2 Moisture and solid content determination (MC and SC): 10 g of the raw garden egg sample was dried in an air drying oven at the temperature of 105 oC for 24 hours [20]. The final weight of the dried sample was recorded. The percentage moisture and solid content was calculated by Equation II and III.
Where Wi and Wf are weight of the garden egg before and after drying respectively while MC is moisture content of the garden egg.
2.4 Experimental
The osmo-dehydration experiments were performed in batches at different agitation time, salt solution concentration and solution temperature in order to understudy the effect of temperature, time and concentration on %water loss (%WL), %solid gain (%SG) and %weight reduction (%WR) of the garden egg. The %WL, %SG and %WR were calculated by Equation IV, V and VI.
Where Wa is the initial mass of fresh sample (g), Wb is the mass of sample after time (t) of osmotic dehydration (g), Wc is the solid gain, Wd is the oven dry mass of sample (g) after time (t) of osmotic dehydration and We is the initial dry mass of sample (g).
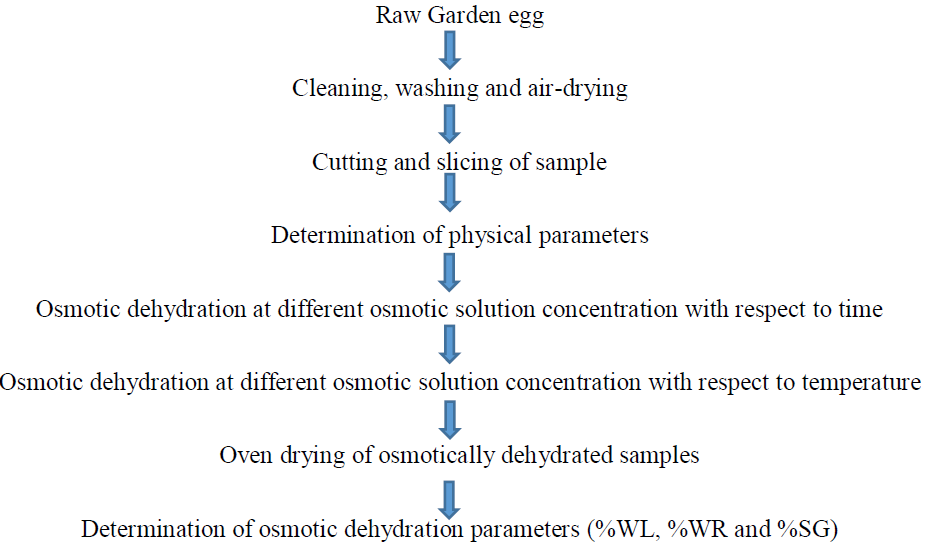
Figure 1: Process flow chart of the garden slices.
2.4.1 Effect of osmotic solution concentrations and osmo-dehydration time on osmotic dehydration of the garden egg slices: The osmotic dehydration experiments were performed at temperature of 40 ºC using a water bath equipped with an incubation system. The experiments were performed in batch at time intervals of 20, 40, 60, 80, 100, 120, 140, 160 and 180 min using 50 mL osmotic solution of different concentration (20, 40 and 60% w/w). At the end of the batch experiment, the samples were blotted with cotton wool to remove excess water from the surface. Thereafter, the samples were weighed in order to determine the % WR of the garden egg slices. Following this, the samples were dried in an air drying oven at the temperature of 105 oC for 24 hrs in order to determine the actual %WL and %SG by the garden egg slices [21].
2.4.2 Effect of osmotic solution temperature on osmotic dehydration of the garden egg slices: The effect of osmotic solution temperature was studied using 10 g of the garden egg slices in different solution concentrations of 20, 40 and 60% w/w. Each sample in each osmotic solution was agitated for a period of 180 min at the temperatures of 25, 35, 45, 55 and 65 oC. Weighing and drying were carried out as described in the process above.
3. Results and Discussion
3.1 Physical parameters
The garden egg has a relative density (1.05 g/mL) corresponding to that of pure water (approximately 1.00 g/mL). The percentage moisture content and solid content of the garden egg are 95.00 and 5.00% respectively (Table 1), which implies that the majority of the matter is composed of water (moisture). The high moisture content and low solid content could possibly account for reason the garden egg has a density corresponding to that of pure water. It has also been shown that the larger percentage of eggplant is made up of moisture [17].
Physical parameter |
Values |
Density |
1.05 g/mL |
%Moisture content |
95.00 |
%Solid content |
5.00 |
Table 1: Physical parameters of the garden egg.
3.2 Effect of salt concentration and osmo-dehydration time on osmotic dehydration of the garden egg slices
The solution concentration and dehydration time are important factors that affect SG, WL and WR. %Water loss, %weight reduction and %solid gained by the garden egg slices were estimated at different concentrations of salt solution (20, 40 and 60 %w/w) and time (20, 40, 60, 80, 100, 120, 140, 160 and 180 min) at the temperature of 40 oC. The results are discussed below.
3.2.1 %Solid (salt) gain: The %SG for: 20 %w/w solution concentration are 37, 43, 48, 54, 81, 80, 86, 89 and 93%; 40 %w/w solution concentration are 35, 40, 71, 78, 85, 100, 110, 118 and 126%; 60 %w/w solution concentrations are 60, 62, 86, 90, 102, 108, 113, 139 and 157%. The results are shown in (Figure 2 and Table 2), while the mean %SG is shown in (Figure 3). This implies that %SG by the garden egg slices for all the concentrations (20, 40 and 60 %w/w) increases with increase in dehydration time from 20 to 180 min. Thus, solid gained by the garden egg with concentration change was time dependent.

Figure 2: % Solid gain with increase in time at varying salt concentration.
Concent-ration (%w/w) |
Time (min) |
Weight after osmo-dehydration (g) |
Weight after oven drying (g) |
%water loss |
Solid gain (g) |
%solid gain |
%weight reduction |
||
20 |
8.41 |
0.87 |
19.60 |
0.37 |
37.00 |
15.90 |
|||
40 |
8.95 |
0.93 |
19.80 |
0.43 |
43.00 |
10.50 |
|||
% 20 |
60 |
8.75 |
0.98 |
22.30 |
0.48 |
48.00 |
12.50 |
||
Salt |
80 |
8.63 |
1.04 |
24.10 |
0.54 |
54.00 |
13.70 |
||
100 |
8.24 |
1.30 |
30.70 |
0.81 |
81.00 |
17.60 |
|||
MEAN SD |
120 140 160 180 |
8.02 7.88 7.76 7.50 |
1.31 1.36 1.39 1.43 |
22.80 29.80 31.30 34.30 26.08 5.48 |
0.80 0.86 0.89 0.93 0.67 0.21 |
80.00 86.00 89.00 93.00 67.89 20.78 |
19.80 21.20 22.40 25.00 17.62 4.87 |
||
20 |
8.62 |
0.85 |
17.30 |
0.35 |
35.00 |
13.80 |
|||
40 |
8.50 |
0.90 |
19.00 |
0.40 |
40.00 |
15.00 |
|||
% 40 |
60 |
8.24 |
1.21 |
24.70 |
0.71 |
71.00 |
17.60 |
||
Salt |
80 |
7.90 |
1.28 |
28.80 |
0.78 |
78.00 |
21.00 |
||
100 |
7.60 |
1.35 |
32.50 |
0.85 |
85.00 |
24.00 |
|||
MEAN SD |
120 140 160 180 |
7.48 7.20 6.91 6.83 |
1.50 1.61 1.68 1.76 |
35.20 39.00 42.70 44.30 31.50 9.82 |
1.00 1.10 1.18 1.26 0.85 0.32 |
100.00 110.00 118.00 126.00 84.78 32.34 |
25.20 28.00 30.90 34.70 23.35 7.17 |
||
20 |
8.54 |
1.10 |
20.60 |
0.60 |
60.00 |
14.60 |
|||
40 |
8.22 |
1.12 |
24.00 |
0.62 |
62.00 |
17.80 |
|||
% 60 |
60 |
8.00 |
1.36 |
28.60 |
0.86 |
86.00 |
20.00 |
||
Salt |
80 |
7.86 |
1.40 |
30.40 |
0.90 |
90.00 |
21.40 |
||
100 |
7.61 |
1.52 |
34.10 |
1.02 |
102.00 |
23.90 |
|||
MEAN SD |
120 140 160 180 |
7.45 7.40 7.22 6.99 |
1.58 1.63 1.69 1.71 |
36.30 37.30 41.70 45.80 33.20 8.14 |
1.08 1.13 1.39 1.57 1.01 0.32 |
108.00 113.00 139.00 157.00 101.89 32.24 |
25.50 26.00 27.80 30.10 23.01 4.98 |
||
Table 2: Osmo-dehydration parameters with respect to time and concentration.
The results from this study showed that the %SG during this process was highest (157%) for the solution with the highest salt concentration (60 %w/w) and higher (126%) for 40 %w/w sucrose solution than that of 20 %w/w salt solution (93%). Therefore, the order of %SG by the garden egg with respect to time is 60 > 40 > 20%. This trend could be attributed to the fact that when the transport of solid (salt) between the fruit’s external surface film and internal pores are equal, the trans–boundary movement of the solid will not be significantly permissible; however, increasing concentration could re-initiate the trans–boundary movement (depending on the nature of the solid and fruit) and hence, the osmotic dehydration process would be concentration dependent. The mean values of the %SG in (Figure 3) also showed that there was a linear relationship between %SG and concentration change during the osmotic dehydration process.
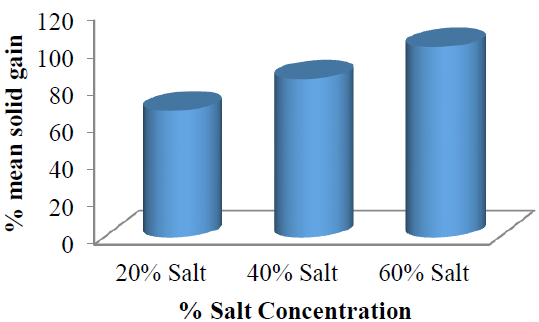
Figure 3: Mean solid (salt) gained by the garden egg at each salt concentration with respect to time.
The results of this study further showed that a higher concentration of salt solution would imply a higher concentration of salt particles to be fixed on the surface and in the pores of the garden egg (i.e. voids created by the osmotically removed water). Filling of air spaces with hypertonic solution of the salt may have resulted to subsequent binding of the solute to the surface of the osmotically dehydrated garden egg thereby resulting to solid gain and increased weight of the actual matter. These findings confirmed that high concentration of salt solution (>50 %w/w) is a mass transfer rate limiting parameter during osmotic dehydration process [22]. Thus, increased salt concentration has significant effect on solid gained. These results corroborate to those obtained by study on osmotic dehydration of mango slices [23].
3.2.2 %Water loss: The most important parameter to examine in osmo-dehydration process is the water loss. It is expected that during a dehydration process, water is lost. The amounts of water loss by the garden egg expressed in percentages are shown in (Figure 4 and Table 2), while the mean %WL is shown in (Figure 7). The %WL for: %20 w/w solution concentrations are: 19.60, 19.80, 22.30, 24.10, 30.70, 22.80, 29.80, 31.30 and 34.30%; 40 %w/w solution concentrations are: 17.30, 19.00, 24.70, 28.80, 32.50, 35.20, 39.00, 42.70 and 44.30% while that of 60 %w/w solution concentrations are 20.60, 24.00, 28.60, 30.40, 34.10, 36.30, 37.30, 41.70 and 45.80%. The results from this study showed that the amount of water removed during this process was highest (45.80%) for solution with the highest salt concentration (60 %w/w) and higher (44.30%) for %40 w/w salt solution than that of 20 %w/w salt solution (34.30%).
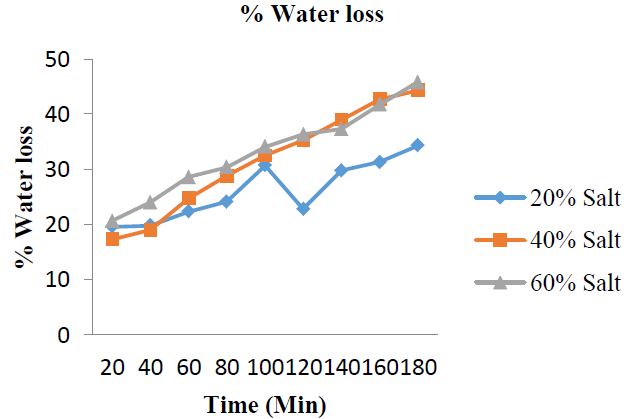
Figure 4: % Water loss with increase in time at varying salt concentration.
These results showed that as the osmo-dehydration time increases, the amount of %WL also increased. This stretched that there was a linear relationship between %WL and osmo-dehydration time of the garden egg. A study by Lewicki and Pawlak [24] also showed that water loss during osmotic dehydration process increased with increase in dehydration time. Also, as the concentration of salt increases, the %WL increases. This also showed linearity of the %WL with increased concentration of salt solution. These results were further supported by the mean values for water loss as represented in (Figure 5).
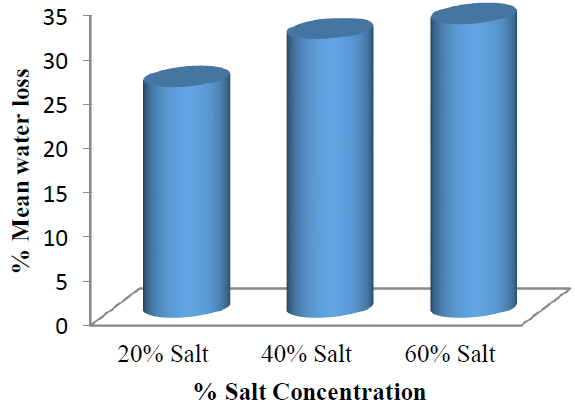
Figure 5: Mean %water loss by the garden egg at each salt concentration with respect to time.
The loss of water could be attributed to increase in concentration of the surrounding medium of the garden egg. This increase in concentration, thereby results into cell shrinkage of the garden egg by plasmolysis with loss of shape of cellular walls together with loss of turgor pressure. Prinzivalli et al. [25] studied the osmotic dehydration of strawberry and their results showed that as water was being loss, there was a gradual disconnection and breakdown of the tissue, with a loss of shape of cellular walls together with loss of turgor pressure during the process. The results of this study are also in line with previously reported work on osmo-dehydration of fruits by Ganjloo et al [26].
3.2.3 %Weight reduction: The results of this study (Figure 6 and Table 2) present the weight reduction of the garden egg samples in percentages. The %WR for 20; 40 and 60 %w/w solution concentrations: 15.90, 10.50, 12.50, 13.70, 17.60, 19.80, 21.20, 22.40, 25.00%; 13.80, 15.00, 17.60, 21.00, 24.00, 25.20, 28.00, 30.90, 34.70% and 14.60, 17.80, 20.00, 21.40, 23.90, 25.50, 26.00, 27.80, 30.10%; respectively. These results showed that %WR of the garden egg slices for all the concentrations (20, 40 and 60 %w/w) increases with increase in dehydration time from 20 to 120 min. This implies a linear relationship between %WR of the garden egg and osmo-dehydration time. Also, the results showed that %WR was highest (34.70%) for 40 %w/w salt solution, followed by 60 %w/w (30.10) then 20 %w/w (25%) salt solution which means that weight reduction is not linearly dependent on increase in salt concentration (Figure 6).

Figure 6: % Weight reduction with increase in time at varying salt concentration.
The mean weight reduction as represented in (Figure 7) also showed the non-linear relationship between weight reduction and increased solution concentration. The non-linearity between these osmotic-dehydration variables may have been as a result of the amount of water lost and solid gained during the process. The %WR with respect to concentration changes of the solution are all in the order of 40 > 60 > 20 %w/w.

Figure 7: Mean weight reduction of the garden egg at each salt concentration with respect to time.
Thus, our study showed that higher concentration of the salt solution may not imply higher amount of weight lost or reduced during osmotic dehydration process of the garden egg as the difference in magnitude of water loss to solid gain could affect the total weight lost. Lewicki and Lukaszuk [27] stated that weight lost in fruits during osmotic dehydration process could be attributed to substantial changes in cellular tissue and cell plasmolysis. Therefore, weight loss of the garden egg may have been influenced by the degradation of the middle lamellae during osmosis and cell plasmolysis during the osmotic dehydration processes. Similar observations were reported by Castello et al [28] on osmotic dehydration of strawberries.
3.3 Effects of solution temperature on osmotic dehydration of garden egg slices
The solution temperature is also an important factor that affects SG, WL and WR. Effects Water loss, weight reduction and solid gain by the garden egg slices using osmotic solution of varying concentrations were studied at different temperature (25, 35, 45, 55 and 65 oC) for the duration of 180 min. The results are shown in (Table 3).
Concent-ration (%w/w) |
Temperature (oC) |
Weight after osmo-dehydration (g) |
Weight after oven drying (g) |
% water loss |
Solid gain (g) |
%solid gain |
%weight reduction |
25 |
8.72 |
1.31 |
20.90 |
0.81 |
81.00 |
12.80 |
|
% 20 |
35 |
8.01 |
1.40 |
28.90 |
0.90 |
90.00 |
19.90 |
Salt |
45 |
7.77 |
1.53 |
32.60 |
1.03 |
103.00 |
22.30 |
MEAN SD |
55 65 |
7.33 7.75 |
1.60 1.61 |
37.70 33.60 30.74 6.33 |
1.10 1.11 0.99 0.13 |
110.00 111.00 99.00 13.09 |
26.70 22.50 20.84 5.12 |
25 |
8.68 |
1.11 |
19.30 |
0.61 |
61.00 |
13.20 |
|
% 40 |
35 |
7.56 |
1.38 |
33.20 |
0.88 |
88.00 |
24.40 |
Salt |
45 |
6.70 |
1.66 |
44.60 |
1.16 |
116.00 |
33.00 |
MEAN SD |
55 65 |
5.30 6.33 |
1.70 1.68 |
49.00 48.50 30.93 17.89 |
1.20 1.18 1.01 0.25 |
120.00 118.00 100.60 25.71 |
37.00 36.70 28.86 10.13 |
25 |
7.75 |
1.39 |
31.40 |
0.89 |
89.00 |
22.50 |
|
% 60 |
35 |
7.02 |
1.55 |
40.30 |
1.05 |
105.00 |
29.80 |
Salt |
45 |
6.50 |
1.78 |
47.80 |
1.28 |
128.00 |
35.00 |
MEAN SD |
55 65 |
6.11 5.99 |
1.82 1.83 |
52.11 53.11 30.04 19.67 |
1.32 1.33 1.17 0.20 |
132.00 133.00 117.40 19.55 |
38.90 40.10 33.26 7.23 |
Table 3: Osmo-dehydration parameters with respect to temperature and concentration.
3.3.1 %Water loss: The results obtained for water loss by the garden egg with respect to temperature change expressed in percentages are shown in (Figure 8 and Table 3), while the mean %WL is shown in (Figure 10). The %WL for: %20 w/w solution concentrations are 20.90, 28.90, 32.69, 37.70 and 33.60%; 40 %w/w solution concentrations are: 19.30, 33.20, 44.60, 49.00 and 48.50%; 60 %w/w solution concentrations are: 31.40, 40.30, 47.80, 52.11 and 53.11% after osmo-dehydration. These results showed that %WL by the garden egg slices for solution concentrations of 20 and 40 %w/w increased with increase in dehydration temperature from 25 to 55 oC and reduced at 60 oC while that of 60 %w/w increased from 25 to 65 oC. Also, the results showed highest %WL (53.11%) by the garden egg for salt solution with concentration of 60% w/w at the temperature of 65 oC, higher %WL (49.00%) for 40 %w/w salt solution at the temperature of 55 oC, while 20 %w/w salt solution has the least value for %WL (37.70%) at 55oC (Figure 8).
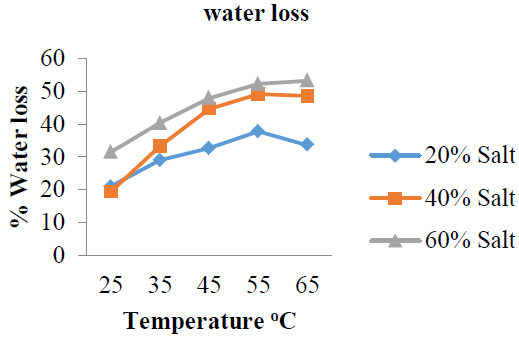
Figure 8: %Water loss at varying temperature.
This implies that temperature solution has significant effect on water loss during osmotic dehydration process of the garden egg. The %WL with respect to concentration changes and temperature of the solution are all in the order of 60 > 40 > 20 %w/w. However, the least mean %WL value was recorded for the salt solution with concentration of 60 %w/w (Figure 9) which could be attributed to the efficiency of the osmotic solution to remove water at the selected temperatures.

Figure 9: Mean %water loss by the garden egg at each concentration with respect to osmo-dehydration temperature.
It has been shown that increase in temperature up to a certain limit is known to increase the rate of osmosis while further increase in temperature affects the semi-permeability of the cell walls and reduces the rate of osmosis [29]. This fact could account for the reason for reduction in water loss at temperature above 55 oC in this study. The results of this study corroborates with previously reported works by Li and Ramaswamy [30].
3.3.2 %Solid (Salt) gain: The %SG by the garden at varying concentrations of the osmotic solution was evaluated at different temperature (25, 35, 45, 55 and 65 oC) for 180 min. The results from the experiments are shown in (Figure 10 and Table 3). The results of solid gain for: %20 w/w solution concentrations are: 81, 90, 103, 110, and 111%; %40 w/w solution concentrations are: 61, 88, 116, 120 and 118%; %60 w/w solution concentrations are: 89, 105, 128, 132 and 133%. These results showed that %SG by the garden egg slices for concentrations of 20 and 60 %w/w increased with increase in dehydration temperature from 25 to 60 oC (Figure 10).

Figure 10: %solid gain at varying temperature.
The increase in %SG from 25 to 65 oC showed the ability of the salt to overcome resistance to mass transfer with increase in kinetic energy to undergo an interaction (surface bonding) with the active sites of the fruit. The %SG by the garden egg slices for concentrations of 40 %w/w increased with increase in dehydration temperature from 25 to 55 oC. The mean %SG as represented in (Figure 11) also showed a linear relationship between %SG and increased solution concentration.

Figure 11: Mean solid (salt) gained by the garden egg at each concentration with respect to osmo-dehydration temperature.
The results corroborate to the results of Castello et al. [28] that worked on the osmotic dehydration of strawberries.
3.3.3 %Weight reduction: The amounts of water loss by the garden egg expressed in percentages are shown in (Figure 12 and Table 3), while the mean %WR is shown in (Figure 13). The somo-dehydration process was carried out for duration of 180 min at the temperature of 25, 35, 45 and 65 oC. The %WR for: %20 w/w solution concentration are: 12.80, 19.90, 22.30, 26.70 and 22.50%; %40 w/w solution concentration are: 13.20, 24.40, 33.00, 37.00 and 36.70%; %60 w/w solution concentration are: 22.50, 29.80, 35.00, 38.90 and 40.10%. These results showed that %weight reduction by the garden egg slices for all the concentrations (20, 40 and 60 %w/w) increased with increase in osmo-dehydration temperature from 25 to 65 oC as shown in (Figure 12 and Table 3).
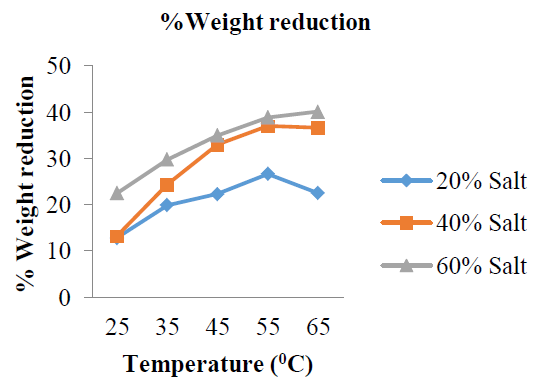
Figure 12: %Weight reduction at varying temperature.
This showed a linear relationship between the osmo-dehydration temperature of the garden egg and concentration of the salt solution. This relationship is further shown in (Figure 13).
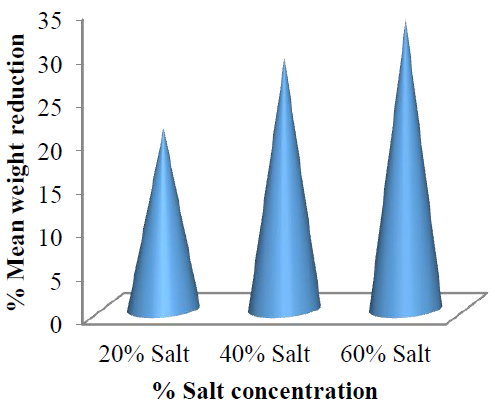
Figure 13: Mean weight reduction of the garden egg at each concentration with respect to osmo-dehydration temperature.
The results therefore showed that weight reduction is temperature dependent. This result is consistent with the study reported by Katsiferis et al [31] on osmotic dehydration of unripe oranges.
4. Conclusion
The results from this study therefore showed that osmotic dehydration by NaCl solution could potentially reduce water activity of garden egg to promote its shelf life or could be employed as a pretreatment technique for further drying, wherefore, desalination (removal of excess salt) and rehydration of dried matter becomes a major advantage of this technique. The data from this study is an added value to the already existing information on conventional techniques for food preservation and osmotic dehydration processes. This study therefore, showed osmotic dehydration process to be a cheap, effective, alternative and beneficial technique for food preservation including garden egg most especially in rural areas.
Conflicts of Interest
The authors declare that there are no conflicts of interest.
References
- Yadav AK, and Singh SV. Osmotic dehydration of fruits and vegetables: a review. Journal of Food, Science and Technology 51 (2014): 1654-1673.
- Heldman DR, Lund, DB. Handbook of Food Engineering, Marcel Dekker, New York (1992).
- Booth IR. Bacterial Responses to Osmotic Stress: Divers mechanisms to Achieve
a Common Goal in “The Properties of Water in Food”. ISOPOW-6 (1998). - Russel NJ, Gould GW. Food Preservatives Blackie Academic and Professional, London (1991).
- Gould GW. New Methods in Food Preservation, Blackie Academic and Professional, London (1995).
- Chenlo F, Chaguri L, Santos F, et al. Osmotic dehydration/impregnation kinetics of padrón pepper (Capsicum annuum Longum) with sodium chloride solutions: process modelling and colour analysis. Food Sci. Technol. Int 12 (2006): 221-227.
- Moreira R, Chenlo F, Torres MD, et al. Effect of stirring in the osmotic dehydration of chestnut using glycerol solutions. LWT – Food Sci. Technol 40 (2007): 1507-1514.
- Matuska M, Lenart A, Lazarides HN. On the use of edible coatings to monitor osmotic dehydration kinetics for minimal solids uptake. J. Food Eng 72 (2006): 85-91.
- Jokie A, Gyura J, Levic L, et al. Osmotic dehydration of sugar beet in combined aqueous solutions of sucrose and sodium J Food Eng 78 (2007): 47-51.
- Ozdemir M, Ozen BF, Dock LL, et al. Optimization of osmotic dehydration of diced green peppers by response surface LWT—Food Sci Technol 41 (2008): 2044-2050.
- Singh B, Panesar PS, Nanda V, et al. Optimization of osmotic dehydration process of carrot cubes in mixtures of sucrose and sodium chloride solutions. Food Chem 123 (2010): 590-600.
- Ferrari CC, Hubinger MD. Evaluation of the mechanical properties and diffusion coefficients of osmodehydrated melon cubes. Int J Food Sci Technol 43 (2008): 2065-2074.
- Moraga MJ, Moraga G, Fito PJ, et al. Effect of vacuum impregnation with calcium lactate on the osmotic dehydration kinetics and quality of osmodehydrated grapefruit. J Food Eng 90 (2009): 372-379.
- Bchir B, Besbes S, Attia H, et al. Osmotic dehydration of pomegranate seeds: mass transfer kinetics and DSC characterization. Int J Food Sci Technol 44 (2009): 2208-2217.
- Mercali GD, Marczak LDF, Tessaro IC, et al. Evaluation of water, sucrose and NaCl effective diffusivities during osmotic dehydration of banana (Musa sapientum, shum.).
LWT—Food Sci Technol 44 (2011): 82-91. - Chen NC, Li HM, Kail T. Eggplant Production, AVRD (2001),
- USDA - United States Department of Agriculture. Eggplant Nutrient Values and Weights for
edible portion. Variability and Change on Food production in Nigeria. 2ndAnnual Conference and Gold (2009): 114-130. - Okito AB, Alves JR, Urquiaga S, et al. Isotopic Fractionation during nitrogen
fixation by four tropical legumes. Soil Biology and Chemistry 36 (2004): 1189-1190. - Dauda SN, Aliyu L, Chiezey, UF. Effect of seedling egg at transplant and poultry
manure on fruit yield and nutrients of garden egg (Solanum gilo ) varieties. Journal of
Tropical Science 5 (2005): 38-41. - Official methods of analysis. Washington: Association of Official Analytical Chemists (1990).
- Najafi H, Yusof YA, Rahman RA, et al. Effect of osmotic dehydration process using sucrose solution at mild temperature on mass transfer and quality attributes of red pitaya (Hylocereus polyrhizusis). International Food Research Journal 21 (2014): 625-630.
- Khoyi MR, Hesari J. Osmotic dehydration kinetics of apricot using sucrose solution. J Food Eng 78 (2007):1355-1360.
- Ito AP, Tonon RV, Park KJ, et al. In?uence of process conditions on the mass
transfer kinetics of pulsed vacuum osmotically dehydrated mango slices. Drying Technology 25 (2007): 1769-1777. - Lewicki PP, Pawlak RP. Effect of osmotic dewatering on apple tissue structure. J Food Eng 66 (2005): 43-50.
- Prinzivalli C, Brambilla A, Maffi D, et al. Effect of osmosis time on structure, texture and pectic composition of strawberry tissue. Eur Food Res Technol
224 (2006): 119-127. - Ganjloo A, Rahman RA, Bakar J, et al. Kinetics Modeling of Mass Transfer Using Peleg’s Equation During Osmotic Dehydration of Seedless Guava (Psidium guajava ): Effect of Process Parameters. Food and Bioprocess Technology 5 (2011): 2151-2159.
- Lewicki PP, Lukaszuk A. Effect of osmotic dewatering on rheological properties of apple subjected to convective drying. Journal of Food Engineering 45 (2000): 119-126.
- Le Maguer M. Osmotic dehydration: review and future directions. Proceedings from International Symposium. On progress in food preservation organized by CERIA, Centre of Education and Research on Food and Chemical Industries, Brussels, Belgium (1988): 12-14.
- Heping L, Hosahalli SR. Osmotic Dehydration: Dynamics of Equilibrium and Pseudo-Equilibrium Kinetics. International Journal of Food Properties 13 (2010): 234-250.
- Castello ML, Fito PJ, Chiralt A. Changes in respiration rate and physical properties of strawberries due to osmotic dehydration and storage. J Food Eng 97 (2010): 64-71.
- Katsiferis T, Zogzas N, Karathanos VT. Mechanical properties and structure of unripe oranges during processing of spoon sweets. Journal of Food Engineering 89 (2008): 149-155.