Measurement of Low Avidity, Polyreactive Immunoglobulin G Antibodies with Increased Sensitivity by Using Low Ionic Strength Buffers
Article Information
Andrea Engelmaier1, Harald Arno Butterweck2, Alfred Weber2*
1Baxalta Innovations GmbH, a Takeda company, Pharmaceutical Sciences, Analytical Development, Vienna, Austria
2Baxalta Innovations GmbH, a Takeda company, PDT R&D, Vienna, Austria
*Corresponding Author: Alfred Weber, Baxalta Innovations GmbH, a Takeda company, PDT R&D, Vienna, Austria
Received: 25 April 2022; Accepted: 03 May 2022; Published: xx May 2022
Citation:
Andrea Engelmaier, Harald Arno Butterweck, Alfred Weber. Measurement of Low Avidity, Polyreactive Immunoglobulin G Antibodies with Increased Sensitivity by Using Low Ionic Strength Buffers. Journal of Analytical Techniques and Research 4 (2022): 71-88.
Share at FacebookAbstract
Low avidity polyreactive immunoglobulin G (IgG) antibodies have a broad range of affinity with dissociation constants of 10-5 to 10-8. Both their low concentration and avidity require low dilutions for measurement in solid-phase assays, which can cause issues due to the high total IgG levels probably interacting with the solid support. Here, we show that using an assay buffer system with low conductivity obviously increases the sensitivity of direct ELISAs. The antigens amyloid-β (Aβ) 40 and Aβ42 peptide, Aβ40 oligomers, Aβ42 fibrils, DNA, tubulin, and thyroglobulin, diluted in 0.1 M carbonate buffer, pH 9.5, were coated to the wells of polystyrene plates. Human citrated normal plasma or the intravenous IgG preparation GAMMAGARD LIQUID was diluted in phosphate-buffered saline or 20 mM HEPES, pH 7.4. Both buffers contained 10 mg/mL human serum albumin. Serial dilution series were loaded to antigen-coated and blank wells after blocking the plate with the respective dilution buffer. Detection of binding was achieved by an anti-human IgG peroxidase and peroxidase staining. Reduced dilution buffer’s conductivity increased signals by at least 50-fold without affecting the binding selectivity. Within the NaCl concentration range from 0 to 150 mM in 20 mM HEPES buffer, the conductivity correlated well with the signal height (R²=0.98). Competition experiments confirmed the assay’s adequate selectivity. In summary, buffer systems with low conductivity significantly increase the signals of direct ELISA without negatively affecting their selectivity. This simple assay modification increases the validity of results obtained by direct ELISAs for measurement of autoantibodies.
Keywords
Autoantibody measurement, Direct ELISA, Assay sensitivity, Low avidity IgGs
Autoantibody measurement articles; Direct ELISA articles; Assay sensitivity articles; Low avidity IgGs articles
Autoantibody measurement articles Autoantibody measurement Research articles Autoantibody measurement review articles Autoantibody measurement PubMed articles Autoantibody measurement PubMed Central articles Autoantibody measurement 2023 articles Autoantibody measurement 2024 articles Autoantibody measurement Scopus articles Autoantibody measurement impact factor journals Autoantibody measurement Scopus journals Autoantibody measurement PubMed journals Autoantibody measurement medical journals Autoantibody measurement free journals Autoantibody measurement best journals Autoantibody measurement top journals Autoantibody measurement free medical journals Autoantibody measurement famous journals Autoantibody measurement Google Scholar indexed journals Direct ELISA articles Direct ELISA Research articles Direct ELISA review articles Direct ELISA PubMed articles Direct ELISA PubMed Central articles Direct ELISA 2023 articles Direct ELISA 2024 articles Direct ELISA Scopus articles Direct ELISA impact factor journals Direct ELISA Scopus journals Direct ELISA PubMed journals Direct ELISA medical journals Direct ELISA free journals Direct ELISA best journals Direct ELISA top journals Direct ELISA free medical journals Direct ELISA famous journals Direct ELISA Google Scholar indexed journals Assay sensitivity articles Assay sensitivity Research articles Assay sensitivity review articles Assay sensitivity PubMed articles Assay sensitivity PubMed Central articles Assay sensitivity 2023 articles Assay sensitivity 2024 articles Assay sensitivity Scopus articles Assay sensitivity impact factor journals Assay sensitivity Scopus journals Assay sensitivity PubMed journals Assay sensitivity medical journals Assay sensitivity free journals Assay sensitivity best journals Assay sensitivity top journals Assay sensitivity free medical journals Assay sensitivity famous journals Assay sensitivity Google Scholar indexed journals Low avidity IgGs articles Low avidity IgGs Research articles Low avidity IgGs review articles Low avidity IgGs PubMed articles Low avidity IgGs PubMed Central articles Low avidity IgGs 2023 articles Low avidity IgGs 2024 articles Low avidity IgGs Scopus articles Low avidity IgGs impact factor journals Low avidity IgGs Scopus journals Low avidity IgGs PubMed journals Low avidity IgGs medical journals Low avidity IgGs free journals Low avidity IgGs best journals Low avidity IgGs top journals Low avidity IgGs free medical journals Low avidity IgGs famous journals Low avidity IgGs Google Scholar indexed journals solid-phase assays articles solid-phase assays Research articles solid-phase assays review articles solid-phase assays PubMed articles solid-phase assays PubMed Central articles solid-phase assays 2023 articles solid-phase assays 2024 articles solid-phase assays Scopus articles solid-phase assays impact factor journals solid-phase assays Scopus journals solid-phase assays PubMed journals solid-phase assays medical journals solid-phase assays free journals solid-phase assays best journals solid-phase assays top journals solid-phase assays free medical journals solid-phase assays famous journals solid-phase assays Google Scholar indexed journals polyreactivity articles polyreactivity Research articles polyreactivity review articles polyreactivity PubMed articles polyreactivity PubMed Central articles polyreactivity 2023 articles polyreactivity 2024 articles polyreactivity Scopus articles polyreactivity impact factor journals polyreactivity Scopus journals polyreactivity PubMed journals polyreactivity medical journals polyreactivity free journals polyreactivity best journals polyreactivity top journals polyreactivity free medical journals polyreactivity famous journals polyreactivity Google Scholar indexed journals immunomodulatory roles articles immunomodulatory roles Research articles immunomodulatory roles review articles immunomodulatory roles PubMed articles immunomodulatory roles PubMed Central articles immunomodulatory roles 2023 articles immunomodulatory roles 2024 articles immunomodulatory roles Scopus articles immunomodulatory roles impact factor journals immunomodulatory roles Scopus journals immunomodulatory roles PubMed journals immunomodulatory roles medical journals immunomodulatory roles free journals immunomodulatory roles best journals immunomodulatory roles top journals immunomodulatory roles free medical journals immunomodulatory roles famous journals immunomodulatory roles Google Scholar indexed journals cytokines articles cytokines Research articles cytokines review articles cytokines PubMed articles cytokines PubMed Central articles cytokines 2023 articles cytokines 2024 articles cytokines Scopus articles cytokines impact factor journals cytokines Scopus journals cytokines PubMed journals cytokines medical journals cytokines free journals cytokines best journals cytokines top journals cytokines free medical journals cytokines famous journals cytokines Google Scholar indexed journals human serum albumin articles human serum albumin Research articles human serum albumin review articles human serum albumin PubMed articles human serum albumin PubMed Central articles human serum albumin 2023 articles human serum albumin 2024 articles human serum albumin Scopus articles human serum albumin impact factor journals human serum albumin Scopus journals human serum albumin PubMed journals human serum albumin medical journals human serum albumin free journals human serum albumin best journals human serum albumin top journals human serum albumin free medical journals human serum albumin famous journals human serum albumin Google Scholar indexed journals intravenous immunoglobulin articles intravenous immunoglobulin Research articles intravenous immunoglobulin review articles intravenous immunoglobulin PubMed articles intravenous immunoglobulin PubMed Central articles intravenous immunoglobulin 2023 articles intravenous immunoglobulin 2024 articles intravenous immunoglobulin Scopus articles intravenous immunoglobulin impact factor journals intravenous immunoglobulin Scopus journals intravenous immunoglobulin PubMed journals intravenous immunoglobulin medical journals intravenous immunoglobulin free journals intravenous immunoglobulin best journals intravenous immunoglobulin top journals intravenous immunoglobulin free medical journals intravenous immunoglobulin famous journals intravenous immunoglobulin Google Scholar indexed journals
Article Details
1. Introduction
Antibodies to foreign and self-antigens [1], which are present without previous immunization and often characterized by a high degree of polyreactivity [2], are part of a human’s natural antibody repertoire. Therefore, they are also termed naturally occurring antibodies or natural autoantibodies [3]. Most of these antibodies, which do not undergo affinity maturation [4], belong to the IgG isotype, display lower affinity and avidity for their antigens, and have more flexible antigen-binding sites than monovalent affinity-maturated antibodies. In addition to certain immunomodulatory roles, involving binding to cytokines and regulation of T-cell responses [5], modulation of dendritic cells [6] or the control of certain types of B cells [7], these antibodies are thought to serve as an initial defense mechanism against pathogens [8]. Their binding repertoire targets peptide [9-13] or carbohydrate epitopes [14-18] presented by self-antigens or nucleic acids [19]. Furthermore, autoantibodies have been implicated in the life cycle of granulocytes [20] and seem to be important for the removal of senescent red blood [21] and apoptotic cells in general [22]. The presence of autoantibodies has often been associated with disease, as reviewed by Ahmed for bleeding disorders [23], and for other disorders as well [24,25]. Disease-associated autoantibodies have been described, for example, in patients with autoimmune hemolytic anemia [26], thrombotic microangiopathies [27], cardiovascular disease [28] or systemic lupus erythematosus [29]. Autoantibodies for neurological diseases, as reviewed by Neff et al. [30] and in particular those targeting β-amyloid in the area of Alzheimer disease [31-34], have gained particular importance. Just recently, prothrombotic autoantibodies [35], autoantibodies that stabilize neutrophil extracellular traps [36], and autoantibodies directed against type I interferons [37] have all been described in severe COVID-19 infections. Generally, autoantibodies may be monovalent or polyreactive and exhibit varying affinities and avidities, with dissociation constants ranging from 10-5 to 10-8 M [38]. Binding of antibodies to their antigens [39] requires close contact between complementary sites: On the one hand, the epitope, provided by the antigen and representing the site, i.e., the antigenic determinant, specifically recognized by the antibody, and on the other hand, the part of the antibody involved in binding, termed a paratope or combining site. With respect to peptide antigens, both interacting sites usually comprise a few amino acids only, either composed of the linear primary amino acid sequence or representing the three-dimensional structure of the antigen. The initial contact between these binding sites is based on electrostatic and hydrophobic forces which have to overcome the repulsion between the two molecules and allow for the expulsion of water molecules as epitope and paratope approach each other. Electrostatic and hydrophobic forces, however, differ in their strength over the distance they are acting. Thus, non-dipole-based ionic interactions decrease with the square of distance, while hydrophobic forces have been shown to exponentially decrease [40]. The bonds subsequently established between the combining site of the antibody and the antigen’s epitope are non-covalent. Participation of hydrogen bonds, electrostatic bonds, or Van der Waals forces has been identified depending on the amino acids involved in the binding. Although all three non-covalent interactions can be considered to be weak, the associations obtained between antigen and antibody can be quite strong but are usually weaker for naturally occurring than for affinity-matured antibodies obtained after immunization. Detection and measurement of specific autoantibodies is useful for the diagnosis of several diseases or providing a risk factor assessment. Direct enzyme-linked immunosorbent assays (ELISAs), where the passively adsorbed antigen of interest is allowed to bind the antibodies from the sample, have often been applied for these purposes. Accurate measurement can be a challenging task, as in some cases the autoantibodies’ polyreactivity is high and their avidity low. We carried out direct ELISAs on a panel of six typical autoantibodies applying low conductivity conditions and demonstrated that the sensitivity clearly increased without loss of selectivity [41].
Abbreviations
Ab, amyloid-b peptide; Ab40, amyloid-b peptide 40; Ab42, amyloid-b peptide 42; CAPS, cross-linked b-amyloid protein species; EC50, equivalence concentration, where half-maximum binding is obtained; ELISA, enzyme-linked immunosorbent assay; GGL, GAMMAGARD LIQUID, Takeda’s licensed intravenous IgG preparation; hSA, human serum albumin; IVIG, intravenous immunoglobulin; OD, optical density; PBS, phosphate-buffered saline; PBST, PBS containing 0.05% Tween 20; RT, room temperature (18 to 25°C); s, conductivity.
2. Material and Methods
2.1 Materials
Maxisorp F96 plates (VWR, Vienna, Austria) were used for the ELISAs. The following antigens were passively adsorbed (= coated) to the plates: Synthetic amyloid-b (Ab)40 and Ab42 peptide (Calbiochem, VWR, Vienna, Austria), cross-linked b-amyloid protein species (CAPS, prepared from Ab40) and Ab42 fibrils. Both Ab conformers were kindly provided by Brian O’Nuallain, Knoxville, Tennessee and were prepared as described [42,43]. DNA (D-8899), tubulin (T-6954) and thyroglobulin (T-1126) were purchased from Sigma (Vienna, Austria) and rabbit anti-human IgG peroxidase (P-0214) from DakoCytomation (Vienna, Austria). Peroxidase staining reagent SureBlue was obtained from KPL (Medac, Hamburg, Germany). The lyophilized citrated reference plasma pool, comprising a minimum of 100 healthy donors, was from Technoclone (Vienna, Austria), human serum albumin (hSA) and eight lots of the intravenous immunoglobulin preparation GAMMAGARD LIQUID (GGL; Lot numbers LE12H252, LE12H253, LE12H254, LE12H255, LE12H256, LE12H302, LE12H303, and LE12H304) were from Takeda Manufacturing Austria (Vienna, Austria). All other chemicals were of the highest analytical grade. In particular, KCl, NaCl, KH2PO4, and Na2HPO4×2 H2O were from VWR (Vienna, Austria) and Tween 20 (EIA grade) was from Bio-Rad (Vienna, Austria).
2.2 Methods
2.2.1 Direct ELISAs: The antigens used were diluted to 5 µg/mL with 0.1 M carbonate buffer, pH 9.5, and coated overnight at 4°C. Blank wells were incubated with 0.1 M carbonate buffer only. Coating was terminated by a washing step using phosphate-buffered saline containing 0.05% Tween 20 (PBST; 0.8% [g/v] NaCl, 0.02% KCl, 0.02% KH2PO4, 0.126% Na2HPO4×2H2O) for the physiological conductivity (sPhys) and 1/10 diluted with water for the low conductivity (sLow) conditions. Similarly, the test samples, which were either a human citrated normal plasma pool or the intravenous immunoglobulin G preparation GAMMAGARD LIQUID, were diluted in 20 mM HEPES, pH 7.2, with/without 150 mM NaCl, but containing 10 mg/mL human serum albumin in both instances. The respective dilution buffers had also been used before loading the samples’ dilutions for blocking the wells using 200 µL/well for 60 min at room temperature (RT). The serial dilution series were loaded to antigen-coated and blank wells (100 µL/well). After an incubation at RT for 2 h, the plates were washed, and bound IgG was detected with a rabbit anti-human IgG peroxidase (100 µL/well, 1 h at RT). After a final washing step, the reaction was stopped with 3 N sulfuric acid (100 µL/well each), and peroxidase measured with SureBlue. The plates were subsequently measured at 450 nm using a reference wavelength of 620 nm. For each dilution, the optical densities (OD) of the blank wells were subtracted from the ODs of the antigen-coated wells to compensate for non-specific binding.
2.2.2 Competition experiments: The antigens DNA and tubulin were used exemplarily for competition experiments to demonstrate the selectivity of the direct ELISAs under low conductivity conditions. The human reference plasma pool sample, diluted in 20 mM HEPES, 20 mM NaCl, pH 7.2, was mixed 1+1 with increasing concentrations of DNA and tubulin ranging from 6 to 50 µg/mL. Immediately after their preparation, the mixtures were loaded to the plate and incubated at RT for 2 h. After a washing step, bound IgG was determined with anti-human IgG peroxidase and SureBlue. Signals obtained in the presence of the antigens were related to those obtained without antigen and the percentage of inhibition was calculated.
2.2.3 Influence of conductivity on signal strength: In a separate experiment, using DNA as antigen, the ionic strength of the dilution buffer was varied from 0 to 120 mM NaCl, adjusting incremental NaCl increases of 20 mM. The direct ELISA for anti-DNA IgG was carried out as described above, using also the ionic strength predefined for the dilution buffer for the respective washing buffer. The binding curves obtained for the serial dilutions series of the plasma sample were evaluated for their equivalence concentration (EC50 values), calculated with GraphPad Prism 6, and providing the half-maximum binding obtained for the respective binding curve.
3. Results
Figure 1 illustrates the overall principle of the direct ELISAs carried out at physiological (sPhys) and low (sLow) conductivity conditions, obtained by just decreasing the NaCl concentrations from 150 mM to zero and running the assay only in the presence of 20 mM HEPES. Of note, the buffer used for the different washing steps during the direct ELISA also had low conductivity, to avoid dissolving the probably labile immune complex formed.
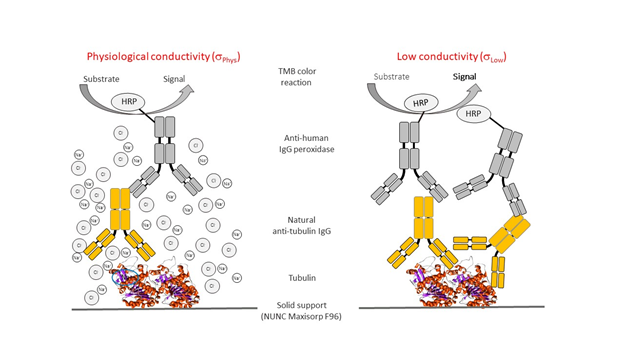
Figure 1: Basic principle of the low conductivity direct ELISA format.
Remarks: Passively adsorbed antigens such as tubulin in the example coated to a NUNC Maxisorp F96 plate are able to capture antibodies present in the test sample. The plate-immobilized antigen-antibody complex will in the next step be detected by using an anti-human peroxidase-labelled IgG-enzyme conjugate, the peroxidase activity of which can easily be measured by using a chromogenic substrate. Operating the direct ELISA at low conductivity conditions, as shown in the right part of the figure, increases its sensitivity without affecting its selectivity because ionic interactions involved in antigen-antibody complex formation are not disrupted.
3.1 Results for anti-AβIgG contained in plasma and the IVIG preparation GGL
Figure 2 shows the binding curves obtained by the direct ELISA for anti-Aβ40 IgG, determined in the human reference plasma pool (panel A) and the IVIG preparation GAMMAGARD LIQUID (panel B).

Figure 2: Direct ELISA binding curves for anti-Aβ40 IgG contained in human plasma and the intravenous IgG preparation GAMMAGARD LIQUID at different conductivities.
Remarks: Disaggregated Aβ40 peptide was passively adsorbed to NUNC Maxisorp F96 plates, while blank wells were prepared by incubation with coating buffer alone. After blocking, the plates were incubated with serial dilutions of a human reference plasma preparation and the IVIG preparation GAMMAGARD LIQUID. Binding curves were established between the IgG concentrations and signals obtained for the Aβ-coated and the blank wells. Two levels of conductivity (σLow and σPhys) were adjusted by using 20 mM HEPES and 20 mM HEPES containing 0.15 M NaCl.
Before the final dilution with coating buffer, the anti-Aβ40 peptide was dissolved in trifluoro acetic acid to disintegrate any higher order Aβ structures possibly present in the lyophilized preparation. Based on our experience from the analysis of 260 healthy plasma donations for anti-Aβ42 IgG [44], where about 25% of the donations showed no specific binding to Aβ peptide but seemed rather to bind to the polystyrene, we trusted in a rigorous blanking approach, loading each dilution to a Aβ40-coated (full symbols) and a blank well (open symbols). Both binding curves are shown. The about 100-times increase in sensitivity is obvious for the binding curves obtained for the reference plasma pool and the IVIG preparation. Applying the low conductivity conditions furthermore enabled us to use higher sample dilutions for the analysis and thus to further decrease the signal obtained on the blank wells, minimizing the non-antigen specific binding. Table 1 shows the results, expressed as OD/mg total IgG, determined for eight GGL lots at both physiological and low conductivity conditions, using plates coated with Aβ42, CAPS, and Aβ fibrils. The result OD/mg total IgG was calculated from the individual dose-response curves obtained. Electrophoresis and electron microscopy confirmed the presence of higher order structures in the CAPS and Aβ-fibril preparations [40,41], while a trifluoro acetic acid treatment immediately before the coating step maintained the monomeric structure of the Aβ42 peptide [42].
Lot No. |
OD/mg total IgG |
||||||||
Aβ42 |
Aβ42 CAPS |
Aβ42 fibrils |
|||||||
sPhys |
sLow |
Increase |
sPhys |
sLow |
Increase |
sPhys |
sLow |
Increase |
|
LE12H252 |
0.72 |
50 |
69 |
1.58 |
73 |
46 |
0.6 |
44 |
73 |
LE12H253 |
1.98 |
65 |
33 |
2.36 |
98 |
41 |
1.22 |
53 |
43 |
LE12H254 |
1.58 |
55 |
35 |
2.07 |
100 |
48 |
1.28 |
55 |
43 |
LE12H255 |
1.91 |
46 |
24 |
2.46 |
87 |
35 |
1.3 |
53 |
41 |
LE12H256 |
2.6 |
63 |
24 |
2.27 |
115 |
51 |
1.58 |
87 |
55 |
LE12H302 |
1.29 |
79 |
62 |
1.55 |
120 |
77 |
1.07 |
64 |
60 |
LE12H303 |
2.21 |
111 |
50 |
2.23 |
140 |
63 |
1.03 |
85 |
82 |
LE12H304 |
1.06 |
108 |
102 |
1.3 |
89 |
69 |
0.4 |
46 |
116 |
Mean |
1.67 |
72 |
50 |
1.98 |
103 |
54 |
1.06 |
61 |
64 |
Table 1: Concentrations of anti-Aβ42, anti-Aβ CAPS and anti-Aβ fibril IgG in the intravenous IgG preparation GAMMAGARD LIQUID measured at two conductivities
Remarks: Anti-Aβ42, anti-CAPS and anti-fibril IgG concentrations were determined on antigen-coated and blank wells using low (σLow) and physiological (σPhys) conductivity conditions. OD differences between coated and blank wells were used to calculate regression lines between signals and total IgG concentrations, expressing the binding activities as OD per mg total IgG.
The rigorous blanking method was also applied. The increases in the anti-Aβ IgG binding observed ranged from 24- to 102-times and from 35- to 77-times for the Aβ42 monomer and CAPS, respectively, and from 41- to 116-times for the Aβ fibrils. Similar mean increases of 50-, 54-, and 64-times were determined for IgG binding to the three Aβ conformers.
3.2 Measurement of other autoantibodies and selectivity confirmation
Figure 3 shows the binding curves for autoantibodies against DNA (panel A), tubulin (panel B), and thyroglobulin (panel C) present in a normal human plasma pool. These curves were obtained at both physiological (σPhys) and low conductivity (σLow) conditions, differentiated by the actual NaCl concentration, i.e., 150 or 0 mM NaCl. Again, the signals obtained on the antigen-coated (full symbols) and the blank wells (open symbols) were related. The binding of naturally occurring anti-DNA IgG increased by a factor of 80 when the assay was carried out at low conductivity. Thus, the OD/mg total IgG increased from 0.038 to 3.0 at decreased conductivity. Similarly, the binding of naturally occurring anti-tubulin IgG increased by a factor of 90 at low conductivity conditions from 0.064 at 0.15 M NaCl to 5.74 OD/mg total IgG at 0 mM NaCl. Finally, the binding of anti-thyroglobulin IgG antibodies also increased by a factor of 78 when the assay was carried out at low conductivity. The OD/mg total IgG values increased from 0.141 to 11.0 when the NaCl concentration was decreased from 150 to 0 mM NaCl. The low conductivity conditions, however, did not impact assay selectivity as shown by the competition experiment (panel D), which was carried out exemplarily for anti-DNA and anti-tubulin IgGs. Addition of the respective antigens to the sample before running the direct ELISA caused dose-dependent signal reductions. Thus, the addition of 50 μg DNA caused almost complete signal reduction (91% inhibition), while lower concentrations resulted in dose-dependent reduced signals. Addition of 50 μg tubulin to a test sample with a total IgG concentration of 250 μg/mL caused significant signal reduction (71% inhibition). However, the inhibition of the antibody binding to a solid phase-immobilized antigen by formation of the antigen-antibody complex in solution requires usually higher amounts of antigen than would correspond to an equimolar level, most probably because the antigen adopts a higher order structure after coating [45]. Nevertheless, dose-dependent inhibition was obtained in both cases, demonstrating the specificity of the binding under the low conductivity conditions as well.

Figure 3: Direct ELISA binding curves for anti-DNA, anti-tubulin and anti-thyroglobulin IgGs in human plasma and competition curves for anti-DNA and anti-tubulin IgG.
Remarks: Panel A and panel B show the direct ELISA binding curves for anti-DNA and anti-tubulin IgGs respectively, obtained on coated and blank wells for a human reference plasma pool, while panel C shows the same data for anti-thyroglobulin IgG. Again, two levels of conductivity (σLow and σPhys) were adjusted by using 20 mM HEPES and 20 mM HEPES containing 0.15 M NaCl. Panel D finally shows the competition curves obtained by incubating the test sample with gradually increasing levels of the antigens DNA and tubulin before the incubation with the antigen-coated wells took place at low conductivity conditions. In particular, antigen concentrations are plotted against the level of signal reduction observed.
3.3 Relation between ionic strength and binding of anti-DNA IgG
The relation between ionic strength and binding of anti-DNA IgG contained in a human normal plasma pool to passively adsorbed DNA was investigated by using 20 mM HEPES buffer, pH 7.2, containing 0 to 120 mM NaCl, in 20 mM increments, and 150 mM NaCl. Table 2 shows the EC50 values calculated for the binding curves together with their 95% confidence intervals and the R² of the fitted curves. There was a clear increase in the EC50 values with the NaCl concentrations from 0.12 to 255.2 µg/mL when the NaCl concentration increased from 0 to 150 mM. All binding curves showed adequate fitting, as demonstrated by the R² of at least 0.99.
NaCl concentration [mM] |
EC50 [µg total IgG/mL] |
Lower 95% confidence interval |
Upper 95% confidence interval |
R² |
0 |
0.12 |
0.11 |
0.13 |
0.9983 |
20 |
0.42 |
0.31 |
0.57 |
0.9926 |
40 |
1.59 |
1.22 |
2.07 |
0.997 |
60 |
7.98 |
6.59 |
9.68 |
0.9951 |
80 |
23.7 |
20.5 |
27.4 |
0.9973 |
100 |
73.9 |
57 |
95.7 |
0.9984 |
120 |
202.2 |
119.7 |
341.5 |
0.9982 |
150 |
255.2 |
181 |
359.8 |
0.9993 |
Table 2: Anti-DNA IgG binding at different ionic strength
Remarks: Curve fitting and calculation of upper and lower 95% confidence interval limits as well as of R² was done with GraphPad Prism 6 using the variable slope four parameter function log analyte (NaCl concentration) versus response (OD).
Figure 4 shows these curves (panel A) and the regression curves between EC50 and NaCl concentrations. The correlation analysis, done between the logarithms of the EC50 values and the NaCl concentrations, resulted in a linear relation for NaCl levels between 0 and 120 mM with an R² of 0.996. This clearly demonstrated that conductivity influences the signal intensity measured in a direct ELISA of naturally occurring anti-DNA IgG in human normal plasma.

Figure 4: Anti-DNA binding curves obtained at different ionic strength.
Remarks: Part A shows the binding curves for anti-DNA IgG obtained in plasma at different ionic strength adjusted in 20 mM HEPES buffer by addition of 0 to 150 mM NaCl. Curve fitting was obtained with GraphPad Prism 6 using the variable slope four parameter function log analyte versus response. Part B shows the regression curve calculated between the NaCl concentrations and the EC50, which indicates the IgG concentration for which half-maximum binding was observed. The error bars mark the 95% confidence intervals determined.
Of note, a double sandwich ELISA for the measurement of the plasma protein β1-antitrypsin [46], based on using antibodies obtained by intentional immunization, did not show any changes in performance when carried out at low conductivity conditions (data on file).
4. Discussion
Detection and measurement of autoantibodies in patients suffering from an autoimmune disease is important because their levels can serve as a diagnostic marker or indicator of disease progression. For instance, rising levels of anti-DNA antibodies in systemic lupus erythematosus correlate with increasing disease activity [47]. Other well-described examples include anti-Aβ in Alzheimer disease, anti-thyroglobulin in thyroiditis, anti-tubulin in autoimmune liver disorders, and rheumatoid factors in rheumatoid arthritis [48]. Clearly, results from an autoantibody assay are only valuable when their level of reliability is acceptable. In the case of anti-DNA autoantibodies, three methods are mainly used for their determination: ELISA, Farr radioimmunoassay and Crithidia luciliae indirect fluorescence antibody. These methods vary in sensitivity and specificity. The Farr assay, for instance, detects only anti-DNA autoantibodies with relatively high avidity, as its procedure includes a precipitation step with high salt concentrations for the immune complexes formed, which causes low avidity complexes formed to dissociate. This particular feature can be judged as disadvantageous because autoantibodies with moderate to low avidity may also have clinical importance. This is obvious in Alzheimer disease, where IgG autoantibodies with low to moderate avidity have been demonstrated to bind to various epitopes presented by different Aβ conformers [49]. Furthermore, the Farr assay applies a radiolabel and is labor intensive like the immune fluorescence assay, which detects autoantibodies with moderate to high avidity. Generally, ELISAs demonstrate higher sensitivity and are amenable to automation. Consequently, they often become the method of choice. Conventional ELISAs, however, also have deficiencies. ELISA measurement can result in widely differing estimates on the relative titers of anti-Aβ IgG in patients with Alzheimer disease versus age-matched controls. While an initial study found lower titers of antibodies against Aβ monomer in patients with Alzheimer disease than in healthy, non-demented controls [50], subsequent studies reported equal or even increased titers of circulating anti-Aβ in patients with Alzheimer disease [51]. In the latter study, anti-Aβ IgG levels were measured after their purification from plasma using Protein G affinity chromatography. This methodology requires the elution of bound IgG by acidic pH, when usually pH 2.5 is applied. The higher anti-Aβ IgG levels found have been interpreted to mirror the presence of anti-Aβ antibodies circulating in complex with Aβ and dissociated by the acidic elution conditions applied so that they became measurable. On the other hand, the strong acidic conditions of pH 2.5 might introduce partial IgG denaturation. Using mouse IgG, Li et al. [52] showed that pH 2.5 elution has three distinct effects: (i) low avidity anti-Aβ IgG (artificially) increased binding, (ii) anti-Aβ IgGs were inactivated time-dependently and (iii) circulating complexes formed between Aβ and high-avidity anti-Aβ IgGs were dissociated. All three reactions were shown to affect the ELISA signals. To overcome these obvious handicaps, they proposed a pH 3.5 elution, which resulted in increased anti-Aβ titers providing an efficient degree of complex dissociation without the negative consequences on IgG nativity observed at the lower pH 2.5. High background binding to empty wells [33,44] and probable interference from other plasma proteins or components present a challenge for the measurement of autoantibodies. Efforts to improve the signal-to-noise ratio and consequently to increase the assay sensitivity have been disclosed for example by Brettschneider el al., who developed a radio-immunoprecipitation assay for the measurement of anti-Aβ IgGs [53]. Another approach to address the same question was proposed by Szabo et al., who tried to remove naturally occurring antibodies binding to polystyrene and other matrices by pre-incubating the samples with those compounds [54]. Although these assay-interfering antibodies were depleted to a certain extent, depletion was not strictly limited to the targeted population of antibodies but also reduced the (real) anti-Aβ titers measured. Use of low sample dilutions is another common practice to compensate for low autoantibody concentrations but this technique does not change the signal-to-noise ratio. More basic issues associated with the robustness of such assays are illustrated by the following example. Anti-prothrombin antibodies have been identified as a hallmark of the autoimmune condition systemic lupus erythematosus and have been detected in about 40% of patients suffering from this disease. Interestingly, the detection of these autoantibodies has been associated with experimental details of their analysis, and not only the use of untreated or γ-irradiated microtiter plates but also, and even more surprisingly, the choice of the assay buffer has been demonstrated to influence the results obtained [55]. Thus, patients’ samples that showed no signal when analyzed on irradiated plates with Tris-buffered saline showed clear positive responses when analyzed after dilution with PBS [56]. Our approach to increase the robustness and sensitivity of such assays was to apply low conductivity conditions during the whole assay. Thus, these conditions favored ionic interactions between antigen and antibody combining sites, which, after initial attraction has taken place, subsequently supports the establishment of other non-covalent linkages based on shorter acting forces such as hydrogen bonds, van der Waals forces or hydrophobic interactions. Katnik et al. reported a similar approach, although they only used it to preserve the labile immune complex [57]. Unlike naturally occurring polyreactive antibodies, antibodies to carbohydrate epitopes are often endowed with a high degree of specificity, allowing the selective recognition and binding to structural isomers of oligosaccharides. The monoclonal antibody termed CSLEX1, binding to the tumor-associated sialyl Lewisx tetrasaccharide Neu5Ac.2→3Galβ1→4[Fuca1→3]GlcNAcβ [58], represents an archetypical example for such highly specific antibodies. Despite its extraordinary specificity, binding was demonstrated to occur at low avidity only, which hampered the development of an adequate ELISA until after the antibody-antigen complex was washed with ten times diluted salt solution to prevent complex dissociation. Obviously, and in line with the strongly charged epitope due to the presence of sialic acid, the antibody-antigen complex formation seems to have included ionic interactions which were enhanced at low ionic strength. Clearly, the use of washing conditions with low ionic strength do not resemble physiological conditions but the given application for the detection of a tumor-associated antigen confirmed the feasibility of this approach. In addition, it has to be considered that repeated epitopes which are often displayed especially by carbohydrate autoantigens will provide an increase in binding clearly outperforming that of the single epitope. Unfortunately, such additive effects, however, cannot be mimicked by direct ELISAs. By contrast, our approach uses rigorous blanking and low conductivity conditions during the whole assay, starting at the moment the sample is diluted and loaded to the plate. As shown for several autoantigens, these conditions considerably (at least 50 times) increased the sensitivity of direct ELISAs without reducing their specificity. Although these beneficial effects of low conductivity conditions are demonstrated here for direct ELISAs only, it seems reasonable to assume that other methodologies, including surface plasmon resonance or high throughput methods such as microarray binding tests, could also benefit from such conditions.
4. Conclusion
The use of buffers with conductivities clearly below the normal physiological range considerably increases the sensitivity of direct ELISAs for the measurement of various low-avidity autoantibodies by increasing initial ionic interactions between antigen and antibody without decreasing their overall specificity.
Acknowledgments
The authors thank Eva Minibeck and Tanja Aleksic for skillful technical assistance, both full time employees of Baxalta Innovations GmbH at the time of the study, Brian O’Nuallain for providing the Aβ conformers and Elise Langdon-Neuner for her editorial support, funded by Baxalta Innovations GmbH. The work was sponsored by Baxalta Innovations GmbH, a Takeda company.
References
- Djoumerska I, Tchorbanov A, Pashovyz A, et al. The autoreactivity of therapeutic intravenous immunoglobulin (IVIg) preparations depends on the fractionation methods used. Scandinavian Journal of Immunology 61 (2005): 357-63.
- Satapathy AK, Ravindran B. Naturally occurring .-galactosyl antibodies in human sera display polyreactivity. Immunology Letters 69 (1999): 347-351.
- Lacroix DS, Kaveri SV, Mouthon L, et al. Self-reactive antibodies (natural autoantibodies) in healthy individuals. J Immunol Methods 216 (1998): 117-137.
- Teng G, Papavasiliou FN. Immunoglobulin Somatic Hypermutation. Annu. Rev. Genet 41 (2007): 107-120.
- Nielsen CH, Bendtzen K. Immunoregulation by naturally occurring and disease-associated autoantibodies: Binding to cytokines and their role in regulation of T-cell responses, in: Naturally occurring antibodies (NAbs), Advances in Experimental Medicine and Biologics 750 (2012).
- Kwekkeboom J. Modulation of dendritic cells and regulatory T cells by naturally occurring antibodies, in: Naturally Occurring Antibodies. Advances in Experimental Medicine and Biologics 750 (2012).
- Pasquali JL, Martin T. Control of B cells expressing naturally occurring autoantibodies, in: Naturally Occurring Antibodies. Advances in Experimental Medicine and Biologics 750 (2012).
- Bouhlal H, Kaveri S. Multi-faceted role of naturally occurring autoantibodies in fighting pathogens, in: Naturally Occurring Antibodies. Advances in Experimental Medicine and Biologics 750 (2012).
- Bernier VF, Rabilloud R, Rousset B. Evidence for anti-tubulin autoantibodies in the form of immune complexes in human sera. Clin exp Immunol 71 (1988): 261-268.
- Gunten S, Schaub A, Vogel M, et al. Immunological and functional evidence for anti-Siglec-9 autoantibodies in intravenous immunoglobulin (IVIg). Blood 108 (2006): 4255-4259.
- Reipert BM, Stellamor MT, Poell M, et al. Variation of anti-Fas antibodies in different lots of intravenous immunoglobulins. Vox Sang 94 (2008): 334-341.
- Sarter K, Janko C, André S, et al. Autoantibodies against galectins are associated with antiphospholipid syndrome in patients with systemic lupus erythematosus. Glycobiology 23 (2012): 12-22.
- Bouhlal H, Martinvalet D, Teillaud JL, et al. Natural autoantibodies to Fcγ receptors in intravenous immunoglobulins. J Clin Immunol 34 (2014): S4-S11.
- Lang GA, Yeaman GR. Autoantibodies in endometriosis sera recognize a Thomsen-Friedenreich-like carbohydrate antigen. Journal of Autoimmunity 16 (2001): 151-161.
- György B, Tóthfalusi L, Nagy G, et al. Natural autoantibodies reactive with glycosaminoglycans in rheumatoid arthritis. Arthritis Research & Therapy 10 (2008): R110.
- Gunten S, Smith D, Cummings R, et al. Intravenous immunoglobulin contains a broad repertoire of anti-carbohydrate antibodies that is not restricted to the IgG2 subclass. J Allergy Clin Immunol 123 (2009): 1268-1276.
- Luetscher RND, McKitrick TR, Gao C, et al. Unique repertoire of anti-carbohydrate antibodies in individual human serum. Scientific Reports 10 (2020): 15436.
- Schwartz-Albiez R. Naturally occurring antibodies directed against carbohydrate tumor antigens, in: Naturally Occurring Antibodies. Advances in Experimental Medicine and Biologics 750 (2012).
- Swanson PC, Ackroyd C, Glick GD. Ligand recognition by anti-DNA autoantibodies. Affinity, specificity, and mode of binding. Biochemistry 35 (1996): 1624-1633.
- Gunten S, Simon HU. Granulocyte death regulation by naturally occurring autoantibodies, in: Naturally Occurring Antibodies. Advances in Experimental Medicine and Biologics 750 (2012).
- Lutz HU. Naturally occurring autoantibodies in mediating clearance of senescent red blood cells, in: Naturally Occurring Antibodies. Advances in Experimental Medicine and Biologics 750 (2012).
- Elkon KB, Silverman GJ. Naturally occurring autoantibodies to apoptotic cells, in: Naturally Occurring Antibodies (NAbs), Advances, in: Experimental Medicine and Biologics 750 (2012).
- Ahmed AEE. Autoantibodies to coagulation factors and bleeding disorders. Clin reviews in Allergy and Immunol 16 (1998): 313-319.
- Cervera R, Shoenfeld Y. Pathogenic mechanism and clinical relevance of autoantibodies, in autoantibodies (2007) Elsevier.
- Prüss H. Autoantibodies in neurological disease. Nature Reviews Immunology 21 (2021): 798-813.
- Fossati JL, Reininger L, Chicheportiche Y, et al. High pathogenic potential of low-affinity autoantibodies in experimental autoimmune hemolytic anemia. J Exp Med 190 (1999): 1689-1696.
- Rieger M, Mannucci PM, Kremer HJA, Herzog A, Gerstenbauer G, Konetschny C, Zimmermann K, Scharrer I, Peyvandi F, Galbusera M, et al. ADAMTS13 autoantibodies in patients with thrombotic microangiopathies and other immunomediated diseases. Blood 106 (2005): 1262-1267.
- Teixeira PC, Ducret A, Ferber P, et al. Definition of human apolipoprotein A-I epitopes recognized by autoantibodies present in patients with cardiovascular diseases. J Biol Chem 289 (2014): 28249-28259.
- Sarter K, Janko C, André S, et al. (2012). Autoantibodies against galectins are associated with antiphospholipid syndrome in patients with systemic lupus erythematosus. Glycobiology 23 (2012): 12-22.
- Neff F, Wei X, Nölker C, et al. Immunotherapy and naturally occurring autoantibodies in neurodegenerative disorders. Autoimmunity Reviews 7 (2008): 501-507.
- Bach JP, Dodel R. Naturally occurring autoantibodies against β-amyloid, in: Naturally Occurring Antibodies (NAbs), Advances in Experimental Medicine and Biologics 750 (2012).
- Kellner A, Matschke J, Bernreuther C, et al. Autoantibodies against β-amyloid are common in Alzheimer’s Disease and help control plaque burden. Ann Neurol 65 (2009): 24-31.
- Szabo P, Relkin N, Weksler ME. Natural human antibodies to amyloid beta peptide. Autoimmunity Reviews 7 (2008): 415-420.
- Welzel AT, Williams AD, McWilliams-Koeppen HP, et al. Human anti-Aβ IgGs target conformational epitopes on synthetic dimer assemblies and the AD brain-derived peptide. PLoS One 7(2012): e50317.
- Zuo Y, Estes SK, Ali RA, et al. Prothrombotic autoantibodies in serum from patients hospitalized with COVID-19. Sci. Transl. Med. 12 (2020): 38-76.
- Zuo Y, Yalavarthi S, Navaz SA, et al. Autoantibodies stabilizes neutrophil extracellular traps in COVID-19. JCI Insight 6 (2021): e150111.
- Bastard P, Gervais A, Le Voyer T, et al. Autoantibodies neutralizing type I IFNs are present in ~4% of uninfected individuals over 70 years old and account for ~20% of COVID-19 deaths. Sci. Immunol 6 (2021): 4340.
- Bozic B, Cucnik S, Kveder T, et al. Affinity and avidity of autoantibodies, in: Autoantibodies (2007), ed. by Shoenfeld Y, Gershwin ME, Meroni PL, Elsevier.
- Reverberi R, Reverberi L. Factors affecting the antigen-antibody reaction. Blood Transfus 5 (2007): 227-240.
- Israelachvili J, Pashley R. The hydrophobic interaction is long range, decaying exponentially with distance. Nature 300 (1982): 341-342.
- Weber A, Engelmaier A, Schwarz HP. Measurement of autoantibodies at low conductivity with increased sensitivity. US 9,075,069 B2 (2015).
- O' Nuallain B, Acero L, Williams AD, et al. Human plasma contains cross-reactive Aβ conformer-specific IgG antibodies. Biochemistry 47 (2008): 12254-12256.
- O' Nuallain B, Williams AD, McWilliams-Koeppen HP, et al. Anti-amyloidogenic activity of IgGs contained in normal plasma. J Clin Immunol 30 Suppl 1 (2010): S37-42.
- Weber A, Minibeck E, Engelmaier A, et al. Anti-β-amyloid (Aβ) IgG levels in plasma of healthy people measured with a reliable immunoassay. New Trends in Alzheimer and Parkinson Related Disorders: ADPD 2009 Collection of Selected free Papers from the 9th International Conference on Alzheimer’s and Parkinson’s Disease ADPD (2009).
- Conradie JD, Govender M, Visser L. ELISA solid phase: partial denaturation of coating antibody yields a more efficient solid phase. J Immunol Methods 59 (1983): 289-299.
- Weber A, Butterweck H, Mais-Paul U, et al. Biochemical, molecular, and preclinical characterization of a double-virus-reduced human butyrylcholinesterase preparation designed for clinical use. Vox Sang 100 (2010): 285-297.
- Riboldi, Gerosa, Moroni G, et al. Anti-DNA antibodies: a diagnostic and prognostic tool for systemic lupus erythematosus? Autoimmunity 38 (2005): 39-45.
- Eggert M, Zettl UK, Neeck G. Autoantibodies in Autoimmune Diseases. Current Pharmaceutical Design 16 (2010): 1634-1643.
- Szabo P, Mujalli DM, Rotondi ML, et al. Measurement of anti-beta amyloid antibodies in human blood. J Neuroimmunology 227 (2010): 167-174.
- Weksler ME, Relkin N, Turkenich R, et al. Patients with Alzheimer disease have lower levels of serum anti-amyloid peptide antibodies than healthy elderly individuals. Exp Gerontology 37 (2002): 943-948.
- Mruthinti S, Buccafusco JJ, Hill WD, et al. Autoimmunity in Alzheimer's disease: increased levels of circulating IgGs binding Abeta and RAGE peptides. Neurobiol Aging 25 (2004): 1023-1032.
- Li Q, Gordon M, Cao C, et al. Improvement of a low pH antigen-antibody dissociation procedure for ELISA measurement of circulating anti-Aβ antibodies. BMC Neuroscience 8 (2007): 22-33.
- Brettschneider S, Morgenthaler NG, Teipel SJ, et al. Decreased serum amyloid β1-42 autoantibody levels in Alzheimer’s Disease, determined by a newly developed immuno-precipitation assay with radiolabeled amyloid β1-42 Peptide. Biol. Psychiatry 57 (2005): 813-816.
- Szabo P, Mujalli, Rotondi, et al. A method for measuring anti-beta amyloid (Aβ) oligomer antibodies in human plasma and intravenous immunoglobulin. Alzheimer’s & dementia Journal 4 (2008): 365
- Matsuura E, Igarashi Y, Yasuda T, et al. Anticardiolipin antibodies recognize b2 glycoprotein I structure altered by interacting with an oxygen modified solid phase surface. J Exp Med 179 (1994): 457-462.
- Matsuda J, Saitoh N, Tsukamoto M, et al. Buffer may be the critical factor in measurement of anti-prothrombin antibody on a gamma-ray-irradiated plate by enzyme-linked immunosorbent assay. Am J Hematol 53 (1996): 242-244.
- Katnik I, Goodarzi MT, Turner GA. An improved ELISA for the determination of sialyl Lewis(X) structures on purified glycoconjugates. Glycoconjugate J 13 (1996): 1043-1047.
- Fukushima K, Hirota M, Terasaki PI, et al. Characterization of sialosylated Lewisx as a new tumor-associated antigen. Cancer Res 44 (1984): 5279-5285.