Gestational Intermittent Hypoxia Programs Hypertensive Response in Female Rat Offspring: Impact of Ovaries
Article Information
Ruolin Song1, Jay S Mishra1, Sri Vidya Dangudubiyyam1, Tracy L Baker1, Jyoti J Watters1, Sathish Kumar1,2,*
1Department of Comparative Biosciences, School of Veterinary Medicine, University of Wisconsin, Madison, WI 53706, USA
2Department of Obstetrics and Gynecology, School of Medicine and Public Health, University of Wisconsin, Madison, WI 53792, USA
*Corresponding author: Sathish Kumar, Associate Professor, Department of Comparative Biosciences and Obstetrics and Gynecology, University of Wisconsin- Madison, 2015, Linden Drive, Madison, WI 53706, USA.
Received: 03 June 2022; Accepted: 14 June 2022; Published: 20 June 2022
Citation:
Ruolin Song, Jay S Mishra, Sri Vidya Dangudubiyyam, Tracy L Baker, Jyoti J Watters, Sathish Kumar. Gestational Intermittent Hypoxia Programs Hypertensive Response in Female Rat Offspring: Impact of Ovaries. Journal of Women’s Health and Development 5 (2022): 185-196.
Share at FacebookAbstract
Obstructive Sleep Apnea (OSA) is a chronic condition frequently observed in pregnant women. We have shown that Gestational Intermittent Hypoxia (GIH), a hallmark of OSA, leads to sex-specific impairment in the endothelium-dependent relaxation response and an increase in blood pressure in adult male but not female rat offspring. The present study tested the hypothesis that functional ovaries normalize GIH-induced hypertensive response in female offspring. Experiments were done in female offspring of pregnant rats exposed to normoxia or GIH (FIO2 21–10.5% from gestational days 10 to 21). Ovariectomy and sham surgery were performed at 5 weeks of age. Pups born to GIH dams were significantly smaller than the controls, but they exhibited catch-up growth and were similar to controls by 5 weeks of age. Ovariectomy significantly exacerbated bodyweight gain to a similar extent in both control and GIH offspring. Marked increases in blood pressure were observed in pre-pubertal GIH offspring compared to controls; however, after puberty, blood pressure in GIH offspring progressively decreased and became normotensive at adulthood. Ovariectomy led to the maintenance of higher blood pressure in post-pubertal GIH offspring with no significant effect in controls. Vascular contractile and relaxation responses were not affected in the GIH and control offspring; however, ovariectomy selectively decreased endothelium-dependent relaxation response along with a decrease in endothelial nitric oxide synthase expression in the GIH offspring. These findings suggest that functional ovaries are crucial in protecting females against GIH-mediated endothelial dysfunction and hypertension in adulthood.
Keywords
Intermittent hypoxia; Pregnancy; Blood pressure; Fetal programming; Endothelium; eNOS
Intermittent hypoxia articles Intermittent hypoxia Research articles Intermittent hypoxia review articles Intermittent hypoxia PubMed articles Intermittent hypoxia PubMed Central articles Intermittent hypoxia 2023 articles Intermittent hypoxia 2024 articles Intermittent hypoxia Scopus articles Intermittent hypoxia impact factor journals Intermittent hypoxia Scopus journals Intermittent hypoxia PubMed journals Intermittent hypoxia medical journals Intermittent hypoxia free journals Intermittent hypoxia best journals Intermittent hypoxia top journals Intermittent hypoxia free medical journals Intermittent hypoxia famous journals Intermittent hypoxia Google Scholar indexed journals Pregnancy articles Pregnancy Research articles Pregnancy review articles Pregnancy PubMed articles Pregnancy PubMed Central articles Pregnancy 2023 articles Pregnancy 2024 articles Pregnancy Scopus articles Pregnancy impact factor journals Pregnancy Scopus journals Pregnancy PubMed journals Pregnancy medical journals Pregnancy free journals Pregnancy best journals Pregnancy top journals Pregnancy free medical journals Pregnancy famous journals Pregnancy Google Scholar indexed journals Blood pressure articles Blood pressure Research articles Blood pressure review articles Blood pressure PubMed articles Blood pressure PubMed Central articles Blood pressure 2023 articles Blood pressure 2024 articles Blood pressure Scopus articles Blood pressure impact factor journals Blood pressure Scopus journals Blood pressure PubMed journals Blood pressure medical journals Blood pressure free journals Blood pressure best journals Blood pressure top journals Blood pressure free medical journals Blood pressure famous journals Blood pressure Google Scholar indexed journals Fetal programming articles Fetal programming Research articles Fetal programming review articles Fetal programming PubMed articles Fetal programming PubMed Central articles Fetal programming 2023 articles Fetal programming 2024 articles Fetal programming Scopus articles Fetal programming impact factor journals Fetal programming Scopus journals Fetal programming PubMed journals Fetal programming medical journals Fetal programming free journals Fetal programming best journals Fetal programming top journals Fetal programming free medical journals Fetal programming famous journals Fetal programming Google Scholar indexed journals Endothelium articles Endothelium Research articles Endothelium review articles Endothelium PubMed articles Endothelium PubMed Central articles Endothelium 2023 articles Endothelium 2024 articles Endothelium Scopus articles Endothelium impact factor journals Endothelium Scopus journals Endothelium PubMed journals Endothelium medical journals Endothelium free journals Endothelium best journals Endothelium top journals Endothelium free medical journals Endothelium famous journals Endothelium Google Scholar indexed journals eNOS articles eNOS Research articles eNOS review articles eNOS PubMed articles eNOS PubMed Central articles eNOS 2023 articles eNOS 2024 articles eNOS Scopus articles eNOS impact factor journals eNOS Scopus journals eNOS PubMed journals eNOS medical journals eNOS free journals eNOS best journals eNOS top journals eNOS free medical journals eNOS famous journals eNOS Google Scholar indexed journals chronic articles chronic Research articles chronic review articles chronic PubMed articles chronic PubMed Central articles chronic 2023 articles chronic 2024 articles chronic Scopus articles chronic impact factor journals chronic Scopus journals chronic PubMed journals chronic medical journals chronic free journals chronic best journals chronic top journals chronic free medical journals chronic famous journals chronic Google Scholar indexed journals Obstructive sleep apnea articles Obstructive sleep apnea Research articles Obstructive sleep apnea review articles Obstructive sleep apnea PubMed articles Obstructive sleep apnea PubMed Central articles Obstructive sleep apnea 2023 articles Obstructive sleep apnea 2024 articles Obstructive sleep apnea Scopus articles Obstructive sleep apnea impact factor journals Obstructive sleep apnea Scopus journals Obstructive sleep apnea PubMed journals Obstructive sleep apnea medical journals Obstructive sleep apnea free journals Obstructive sleep apnea best journals Obstructive sleep apnea top journals Obstructive sleep apnea free medical journals Obstructive sleep apnea famous journals Obstructive sleep apnea Google Scholar indexed journals cardiovascular articles cardiovascular Research articles cardiovascular review articles cardiovascular PubMed articles cardiovascular PubMed Central articles cardiovascular 2023 articles cardiovascular 2024 articles cardiovascular Scopus articles cardiovascular impact factor journals cardiovascular Scopus journals cardiovascular PubMed journals cardiovascular medical journals cardiovascular free journals cardiovascular best journals cardiovascular top journals cardiovascular free medical journals cardiovascular famous journals cardiovascular Google Scholar indexed journals Gestational Intermittent Hypoxia articles Gestational Intermittent Hypoxia Research articles Gestational Intermittent Hypoxia review articles Gestational Intermittent Hypoxia PubMed articles Gestational Intermittent Hypoxia PubMed Central articles Gestational Intermittent Hypoxia 2023 articles Gestational Intermittent Hypoxia 2024 articles Gestational Intermittent Hypoxia Scopus articles Gestational Intermittent Hypoxia impact factor journals Gestational Intermittent Hypoxia Scopus journals Gestational Intermittent Hypoxia PubMed journals Gestational Intermittent Hypoxia medical journals Gestational Intermittent Hypoxia free journals Gestational Intermittent Hypoxia best journals Gestational Intermittent Hypoxia top journals Gestational Intermittent Hypoxia free medical journals Gestational Intermittent Hypoxia famous journals Gestational Intermittent Hypoxia Google Scholar indexed journals
Article Details
1. Introduction
Obstructive sleep apnea (OSA) is a common chronic respiratory disorder during pregnancy. OSA is characterized by repeated obstructions or collapses of the pharyngeal airway during sleep, causing periodic apneas that result in nocturnal intermittent hypoxic episodes. The physiologic, anatomic, and hormonal changes in pregnancy are believed to promote maternal OSA [1], and as such, 26% of pregnant women in the third trimester are reported to suffer from gestational OSA [2-4]. OSA is known to affect the functional outcomes of many organ systems in pregnant women; however, the cardiovascular system is particularly susceptible to intermittent hypoxic episodes. Indeed, previous cohort studies have reported that gestational OSA is associated with increased risks of maternal hypertension [5-7], cardiomyopathy, and congestive heart failure [8]. The mechanisms underlying the increased risk for maternal cardiovascular pathology in OSA patients are unclear, but animal models of intermittent hypoxia mimicking hypoxia-reoxygenation events implicate inflammation and oxidative stress as important mediators [9-12]. Most research focuses on how Gestational Intermittent Hypoxia (GIH) affects the cardiovascular system of mothers, and only scarce evidence is available on how GIH modifies the cardiovascular function of offspring. Studies show that maternal GIH is associated with poor fetal growth, low neonatal birth weight, and shortened telomere lengths in neonates [4]. Additionally, few animal studies show that GIH leads to altered neurodevelopment [13,14] and attenuated respiratory motor responses in offspring [15]. Two other animal studies have suggested a possible link between GIH and increased risk of cardiovascular dysfunction in offspring [16,17]. Our recent studies have demonstrated that GIH results in increased blood pressure with impaired endothelium-dependent vasodilation due to insufficient endothelial nitric oxide synthase (eNOS) activation in adult male offspring, whereas female adult offspring appear to be protected [18]. This suggests that sex hormones may play a role in modulating cardiovascular responses in GIH-exposed adult offspring. Because the female GIH offspring exhibited normal blood pressure in adulthood when maximal ovarian function occurs for this strain [19], we hypothesize that functional ovaries may protect against increases in blood pressure in post-pubertal female GIH offspring. Therefore, we designed the present study to determine (1) whether GIH leads to progressive changes in the blood pressure from pre-pubertal age to adulthood in female offspring and (2) whether the presence of intact ovaries plays an important role in blood pressure control in female GIH offspring. We also investigated the influence of ovaries on vascular contractile and relaxation responses in mesenteric arteries isolated from ovary-intact as well as ovariectomized GIH offspring.
2. Methods
2.1. Animals
All experimental studies were performed with prior approval from the Animal Care and Use Committee at the University of Wisconsin-Madison and conducted per NIH guidelines (NIH Publication No. 85–23, revised 1996). Timed-pregnant Sprague-Dawley rats at Gestational Day (GD) 8 (day of positive plug = GD1) were obtained from Charles River (Wilmington, MA) and housed in a temperature- and light-controlled room (25°C, 12:12-h light/dark cycle). Rats were randomly distributed into GIH and normoxic controls. Rats in the GIH group were placed in an experimental setting specially designed for intermittent hypoxia exposures mimicking OSA [18,20]. The system was setup using a transparent methacrylate box (26 cm long, 18 cm wide, 6 cm high) flushed with air cyclically changing its oxygen content (15 sec at 21% O2, 45 sec to get down to 10.5% O2, and 75 sec at 10.5% O2) mimicking a rate of 15 apneas/h, typical of clinically relevant hypoxia levels in human pregnancies during late gestation [20]. Rats in the control group exposed to room air were placed in similar boxes continuously flushed with alternating normoxic room air at 21% O2 with the same duration and flow rate as the GIH group. The exposures were applied for 8 h/day during the light period (8:00-16:00 h) from GD 10 to 21, with food and water being provided ad libitum.
Dams were allowed for a natural delivery at term, and the birth weights of pups were recorded within 12 h of birth. The pup numbers were adjusted to 10 per dam in both control and GIH litters to ensure equal nutrient access for all offspring (pups with extreme weights were removed). The male-to-female pup ratio remained equivalent after culling, when possible. Only female offspring were used for this study. At 5 weeks of age, baseline blood pressures were non-invasively recorded in female control and GIH offspring. Immediately following this, control and GIH offspring underwent an ovariectomy or a sham surgery (referred to as OVX group and intact group, respectively), as described below. Changes in blood pressure were monitored biweekly up to 15 weeks of age, at which point the rats were euthanized using CO2 inhalation. Blood was collected through the cardiac puncture, and mesenteric arteries were isolated for myograph and mRNA/protein studies.
2.2. Ovariectomy
A subset of offspring at 5 weeks of age were subjected to ovariectomy. The rat offspring were anesthetized with 2% isoflurane and placed in a prone position on a heating plate. An area measuring 4 by 4 cm was shaved cephalic from the iliac crest and disinfected for surgery. Incisions parallel to the midline were made to the posterior of the ribs. The abdominal musculature and peritoneum were navigated via blunt dissection to visualize the ovaries. Ovarian vessels and uterine horns were ligated, and the ovaries were clipped off (OVX group). The abdominal muscular opening was sutured, and the skin incision was then closed with wound clips. For sham surgery, the abdominal musculature and peritoneum were incised and the ovaries were visualized, but not removed (intact group).
2.3. Blood Pressure Measurements
We measured blood pressures biweekly using the CODA tail-cuff blood pressure system (Kent Scientific, Torrington, CT, USA) as described [18]. In brief, rats were placed in restrainers on a warming platform and allowed for a 10-min acclimation. A volume pressure recording cuff and an occlusion cuff were applied to the base of the tail and were inflated and deflated every 90 sec. Mean blood pressures were recorded for 15 cycles. Results from the initial 10 inflation cycles were ignored, and the average obtained from the subsequent 5 cycles was taken as the individual mean blood pressure of the given rat.
2.4. Assessment of Vascular Reactivity
Rats were euthanized by CO2 inhalation, and the mesenteric arcade was excised and immersed in oxygenated Krebs physiological buffer solution (in mM: NaCl, 119; KCl, 4.7; CaCl2, 2.5; MgSO4, 1.17; NaHCO3, 25; KH2PO4, 1.18; EDTA, 0.026; and d-glucose, 5.5; pH 7.4). Mesenteric arteries were cut free of connective tissue into 2-mm rings and mounted on a wire myograph (Danish Myo Technology, Aarhus, Denmark) to record isometric tension. After a 15-min incubation in gassed Krebs buffer at 37°C, the segments were stretched to an internal diameter of 0.9 of L13.3 kPa. Arterial rings were exposed to 80 mM potassium chloride (KCl) until reproducible depolarization-induced contractions were achieved. After this, vascular contractile responses to cumulative additions of phenylephrine (PE) (10−9 − 3×10−5 mol/L), which causes α1-adrenoceptor-induced contractions were determined. To assess endothelium-dependent vasodilation, concentration-dependent relaxation response to acetylcholine (ACh; 10-9–10-5 mol/L) in PE-precontracted arterial rings was performed. To determine endothelium-independent relaxation, concentration-dependent relaxation response to sodium nitroprusside (SNP; 10-10–10-6 mol/L) were examined in PE-precontracted arterial rings. To confirm endothelial integrity, mesenteric arterial rings were precontracted with a submaximal PE concentration, and this was followed by testing for ACh-induced relaxation responses.
2.5. Quantitative Real-time PCR
Total RNA from mesenteric arteries was isolated using TRIzol reagent and purified with an RNeasy clean-up kit (QIAGEN, Inc., Valencia, CA). RNA integrity and concentration were determined using a DS-11 spectrophotometer (DeNovix, Wilmington, DE), and cDNA synthesis was performed using 1 μg of total RNA utilizing an iScript cDNA synthesis kit (Bio-Rad, Hercules, CA). The mRNA levels of eNOS were quantified using a CFX96 real-time thermal cycler (Bio-Rad, Hercules, CA). The specific TaqMan Primers for eNOS (Rn02132634_s1) and β-actin (Rn00667869_m1) were from Thermo Fisher Scientific (Waltham, MA). PCR conditions were 2 min at 50° C and 10 min at 95° C for 1 cycle, then 15 sec at 95° C and 1 min at 60° C for 45 cycles. Results were calculated with the 2-ΔΔCT method and expressed in fold change relative to ovary-intact controls. All reactions were performed in duplicate and normalized to β-actin (internal control).
2.6. Western blotting
Mesenteric arteries were homogenized in an ice-cold Radioimmunoprecipitation Assay (RIPA) buffer (Cell Signaling Technology, Denver, MA, USA) supplemented with a Halt protease and phosphatase inhibitor cocktail (Thermo Scientific). After 20-min lysis, lysates were cleared by centrifugation (14,000 g, 10 min, 4° C) and quantified for protein using a BCA assay kit (Pierce; Thermo Scientific, Waltham, MA, USA). Lysates with 40 ug proteins were mixed with a NuPAGE lithium dodecyl sulfate sample buffer and a reducing agent (Invitrogen; Thermo Scientific, Waltham, MA, USA) and were run through 4%–12% gradient NuPAGE Bis-Tris gels (Invitrogen; Thermo Scientific, Waltham, MA, USA) with electrophoreses at 110 V for 2 h. Subsequently, proteins were transferred onto an Amersham Hybond membrane (GE Healthcare, Chicago, IL, USA) at 20 V for 70 min. Blocking of membranes was done with 5% nonfat milk for 1 h at room temperature. Primary antibody incubations were done at 4° C overnight with antibodies diluted 1:1000 in 5% Bovine Serum Albumin (BSA) for total eNOS (#32027, Cell Signaling Technology, Denver, MA, USA), and β-actin (#4970, Cell Signaling Technology, Denver, MA, USA). Horseradish Peroxidase (HRP)-conjugated secondary antibody incubations were performed for 1 h at room temperature. Blots were developed on film with ECL detection kits (Pierce; Thermo Scientific, Waltham, MA, USA). Using ImageJ software, the band intensity were analyzed and the results were expressed as the ratio of normalized band intensity relative to β-actin.
3. Statistical Analysis
GraphPad Prism (GraphPad, San Diego, CA, USA) was used for all analyses. Cumulative concentration-response curves were fitted to a 4-parameter sigmoid curve for vascular reactivity. Contractile responses to PE were calculated as percent PE-induced maximal contraction and percent KCl-induced contraction. Relaxant responses to ACh were calculated as percent relaxation of PE-induced contraction. Emax (maximal responses) and pD2 values (concentration that produces 50% effect) were obtained using regression analysis. Data from several vascular rings of the same rat were averaged and presented as the datum for 1 rat. All data were shown as mean±SEM. Statistical significance (p<0.05) was assessed by analysis of variance (ANOVA) followed by Dunnet’s post hoc testing or Student's t test, where appropriate.
4. Results
4.1. Birthweight and Growth Rate
The litter size was not significantly different between the control (10.35±0.39 pups/dam) and GIH dams (9.72±0.43 pups/dam). In addition, the male-to-female ratio was not significantly different between the control (1.19±0.17) and GIH groups (1.06±0.21). The birth weight of female pups from GIH dams was significantly lower than in those of control dams (Figure 1 and Table 1). At 5 weeks of age, the body weight of GIH and control offspring did not differ from each other, suggesting that GIH offspring exhibited catch-up growth. After that, the GIH and control offspring maintained a similar growth rate up to 15 weeks of age. Ovariectomies exacerbated bodyweight gain in both GIH and control offspring to a similar extent, such that the ovariectomized GIH and control offspring were significantly heavier than their respective ovary-intact counterparts (Figure 1 and Table 1).

Figure 1: Body weights of control and GIH offspring. Data expressed as mean ± SEM. n=5–6 in each group. *p<0.05 vs. control, ap<0.05 vs. respective ovary intact counterparts. OVX: animals subjected to ovariectomy; intact: animals subjected to sham OVX.
Birth weight |
5 weeks |
15 weeks |
||
Intact |
OVX |
|||
Control |
6.8±0.1 |
142.8±3.6 |
299.0±8.3 |
413.2±11.7a |
GIH |
6.1±0.2* |
141.5±2.9 |
301.5±15.3 |
396.5±19.5a |
Data expressed as mean ± SEM. *p<0.05 vs. control, ap<0.05 vs. respective ovary intact counterparts. OVX: animals subjected to ovariectomy; intact: animals subjected to sham OVX. |
Table 1: Body weights (in grams) of control and GIH offspring.
4.2. Blood pressure
Blood pressure in GIH offspring was significantly higher at 5 weeks of age than in control offspring (Figure 2). After puberty, blood pressure in the GIH offspring progressively decreased and became normotensive by 11 weeks of age. Ovariectomies prevented the marked decrease in blood pressure observed in GIH offspring, such that the blood pressure was maintained at higher levels than in ovariectomized-control offspring. However, ovariectomies did not significantly affect blood pressure in control offspring.

Figure 2: Blood pressures in control and GIH offspring. Mean blood pressure was measured using CODA noninvasive blood pressure system once every two weeks starting from 5 to 15 weeks of age in ovary intact and ovariectomized offspring. Ovariectomies were performed after baseline blood pressure measurements at 5 weeks of age. n=5–6 in each group. *p<0.05 vs. control Intact, ap<0.05 vs. GIH Intact.
4.3. Vascular Contractile Responses
In endothelium-denuded mesenteric arterial rings, vascular contractile responses to KCl (a determination of depolarization-induced contraction) and cumulative concentrations of PE (a measurement of α1-adrenoceptor–induced contraction) were similar between control and GIH offspring with or without ovariectomy (Figures 3A and B).
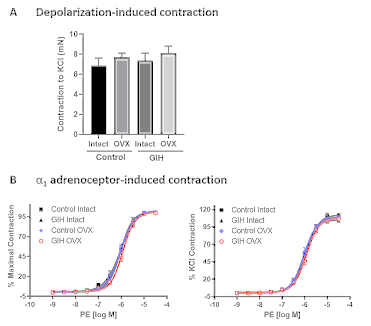
Figure 3: Endothelium-independent vascular contraction in the mesenteric arteries of control and GIH offspring. Mesenteric arterial rings were examined for vascular contractile responses to (A) 80 mM KCl and (B) to cumulative additions of Phenylephrine (PE). PE contractions are expressed as a percentage of maximum PE contraction (left panel) and as a percentage of KCl (80 mM) contraction (right panel). Values are given as means±SEM, with 4–5 rats in each group.
4.4. Vascular Relaxation Responses
In endothelium-intact mesenteric arterial rings, vascular relaxation responses to ACh were similar between GIH (pD2: 7.3±0.04; Emax: 96.4±1.00) and control (pD2: 7.2±0.03; Emax: 99.2±0.22) offspring (Figure 4A). Ovariectomies did not affect ACh-induced relaxation in controls (pD2: 7.1±0.03; ; Emax: 97.5±1.00). In contrast, ovariectomies in GIH offspring led to a significant decrease in ACh-induced relaxation (pD2: 6.6±0.09; Emax: 68.4±8.77) compared to their ovary-intact GIH counterparts (pD2: 7.3±0.04; Emax: 96.4±1.00). In endothelium-intact mesenteric arterial rings, vascular relaxation responses to SNP (an exogenous nitric oxide (NO) donor) were similar between control and GIH offspring with or without ovariectomy (Figure 4B).

Figure 4: Endothelium-dependent and endothelium-independent vascular relaxation in the mesenteric arteries of control and GIH offspring. (A) Endothelium-dependent relaxation responses to cumulative additions of Acetylcholine (ACh) were measured in mesenteric arterial rings precontracted with Phenylephrine (PE). (B) Endothelium-independent relaxation responses to Sodium Nitroprusside (SNP) were measured in PE precontracted mesenteric arterial rings. Values are given as means±SEM, with 4–5 rats in each group.
4.5. eNOS mRNA and Protein Expression
eNOS mRNA and protein expressions were not significantly different between control and GIH offspring (Figures 5A and B). However, ovariectomies significantly decreased eNOS mRNA and protein expression in GIH offspring but not in control offspring (Figures 5A and B).
Figure 5: Endothelial Nitric Oxide Synthase (eNOS) mRNA and protein expression in the mesenteric arteries of control and GIH offspring. mRNA and protein were isolated from mesenteric arteries and subjected to qRT-PCR and Western blotting. Representative western blots are shown on the top. Data are shown as means±SEM, with 4–5 rats in each group. *p<0.05 vs. GIH Intact.
5. Discussion
Our previous study has demonstrated that GIH exposure leads to sex-dependent changes in blood pressure responses in the rat offspring. Female GIH offspring were normotensive in adulthood, while male GIH offspring were hypertensive [18]. The present study provides new information that ovarian function plays a crucial role in protecting females against GIH-mediated developmental programming of hypertension in adulthood. Specifically, the present study offers three major novel findings: (1) GIH resulted in a marked increase in blood pressure response in pre-pubertal female offspring; however, after puberty, their blood pressure was normalized to control levels; (2) ovariectomy had no significant effect on blood pressure response in control offspring, but significantly increased blood pressure in GIH offspring; (3) ovariectomy uncovered GIH-impaired endothelium-dependent relaxation response and decreased eNOS expression in female GIH offspring.
In the present study, we did not monitor maternal food and water consumption, but our previous studies using the same GIH model demonstrated that GIH exposure does not impact maternal food and water intake [18], suggesting that GIH may be directly responsible for the blood pressure and vascular deficits observed in this study. In the present study, we found that GIH significantly decreased the birth weights of term fetuses but not the body weights of adult offspring-findings that are consistent with previous studies [18]. These findings suggest that GIH induces intrauterine growth restriction but promotes catch-up growth in adulthood. However, after ovariectomy, the offspring in the ovariectomized GIH and control groups had significantly higher body weights than their ovary-intact counterparts, suggesting that the presence of functional ovaries may a major factor responsible for the down-regulation of body weight in females, as reported in previous studies [21,22]. In this study, we noted no significant differences in blood pressure responses between female adult ovary-intact control and GIH offspring at 12 to 15 weeks of age, which is consistent with our previous findings that GIH exposure enhances blood pressure response in male but not female offspring in the same animal model [18]. The gender differences in fetal programming of adult hypertension have been reported in other animal models. Most studies indicate that male offspring develop vascular dysfunction, whereas female offspring seem to be protected [22-24]. In the present study, the female GIH offspring developed hypertension at pre-pubertal ages; however, they became normotensive at a post-pubertal age, when maximal ovarian function is attained for this strain [19], suggesting that functional ovaries might protect females from developing hypertension. Further, our finding that ovariectomies facilitated the development of higher blood pressure responses in GIH offspring reaffirms the assertion that functional ovaries contribute to the observed blood pressure differences between pre- and post-pubertal GIH offspring. Overall, these findings indicate that functional ovaries play an important role in protecting female offspring from GIH-induced hypertensive responses. Similar results have been reported in studies with prenatal nicotine exposure and placental insufficiency, wherein the blood pressure was significantly increased following ovariectomy in adverse in utero exposed adult female offspring with no significant effect in adult female control offspring [25,26]. However, how ovaries function to counteract GIH-induced hypertensive response is unclear. It is generally believed that estrogen released from the ovaries may lower arterial blood pressure [27]. In our previous study, using the same model, we found no differences in plasma estradiol levels between the control and GIH offspring. Consistent with our reports, previous studies also indicated that adverse in utero conditions do not affect the circulating estradiol levels in adult female offspring [25, 26]. These findings suggest that the protective role of ovarian function may depend not on estrogen levels per se but on how they affect the downstream regulatory systems that control blood pressure response in adult female GIH offspring. Animal studies show that functional ovaries regulate vascular effects by modulating vascular smooth muscle cells and endothelial cells [28]. Thus, a shift in the vasoconstriction-vasodilation balance may be involved in the susceptibility of ovariectomized GIH offspring to the blood pressure increase. The present study found that depolarization- and α1-adrenoceptor-induced contractions were similar in intact and ovariectomized control and GIH offspring. This finding suggests that ovarian function might not directly regulate vascular contractility to counteract GIH-induced elevated blood pressure responses. On the other hand, we found that ovariectomies significantly decreased ACh-induced relaxations in adult female GIH offspring but had no effect on adult female controls. Thus, the decreased ACh-induced endothelium-dependent relaxation may contribute to the increased blood pressure response in the ovariectomized GIH offspring.
NO is an important factor released from the endothelium and acts on the underlying vascular smooth muscle cells to induce vasodilation. The finding that eNOS mRNA and protein expression were reduced in ovariectomized GIH offspring suggests that NO synthesis is impaired in this group. Further, the observation that relaxation to SNP, an exogenous NO donor, was not significantly different between control and the GIH offspring (with or without ovariectomy) provides evidence that GIH and ovariectomies do not affect the sensitivity of vascular smooth muscle to relaxation. Thus, the reduced ACh-induced relaxation of vascular smooth muscle in ovariectomized GIH offspring is most likely due to a decrease in endothelial NO synthesis. This suggests that ovariectomy may have decreased the ability of the endothelium to release NO and promote vasodilation, leading to increases in blood pressure in GIH offspring. Ovariectomies did not affect blood pressure or vascular eNOS expression in control animals. Therefore, the abnormal response to ovariectomy on blood pressure regulation in adult female GIH offspring may be due to GIH-induced permanent alterations in endothelial function, which is compensated and masked by the presence of functional ovaries. In conclusion, the present study provides novel evidence that functional ovaries play a key role in counteracting GIH-induced vascular endothelial dysfunction and maintaining normotensive responses in post-pubertal female GIH offspring. However, the cellular and molecular mechanisms of how ovaries modify GIH-induced vascular endothelial function, resulting in counteraction of GIH-mediated hypertensive responses, are still unknown. Therefore, future studies are necessary to clarify the mechanisms that initiate the programming of endothelial dysfunction in utero, as well as the signaling interactions between ovaries and vascular endothelial function. Understanding these mechanisms will help develop gender- and age-specific strategies for preventing and treating cardiovascular diseases that originate in utero.
Contribution statement
Sathish Kumar conceptualized and designed the study. Ruolin Song, Jay S. Mishra and Sri Vidya Dangudubiyyam contributed to material preparation, data collection, analysis and interpretation. Tracy L. Baker, Jyoti J. Watters provided scientific input in the experimental design and critically reviewed the manuscript. All authors commented on manuscript revisions and approved the final manuscript.
Conflicts of interest
The authors declare no conflict of interest.
Funding statement
Supported by the National Institutes of Health (NIH) ES033345 and HL134779 (S.K.) and HL142752 (JJW and TLB).
References
- Facco FL, Parker CB, Reddy UM, et al. Association Between Sleep-Disordered Breathing and Hypertensive Disorders of Pregnancy and Gestational Diabetes Mellitus. Obstet Gynecol 129 (2017): 31-41.
- Dominguez JE, Krystal AD, Habib AS. Obstructive Sleep Apnea in Pregnant Women: A Review of Pregnancy Outcomes and an Approach to Management. Anesth Analg 127 (2018): 1167-1177.
- Facco FL, Ouyang DW, Zee PC, et al. Sleep-disordered breathing in a high-risk cohort prevalence and severity across pregnancy. Am J Perinatol 31 (2014): 899-904.
- Dominguez JE, Street L, Louis J. Management of Obstructive Sleep Apnea in Pregnancy. Obstet Gynecol Clin North Am 45 (2018): 233-247.
- Lockhart EM, Ben Abdallah A, Tuuli MG, et al. Obstructive Sleep Apnea in Pregnancy: Assessment of Current Screening Tools. Obstet Gynecol 126 (2015): 93-102.
- O'Brien LM, Bullough AS, Owusu JT, et al. Pregnancy-onset habitual snoring, gestational hypertension, and preeclampsia: prospective cohort study. Am J Obstet Gynecol 207 (2012): 487.e1-9.
- Pérez-Chada D, Videla AJ, O'Flaherty ME, et al. Snoring, witnessed sleep apnoeas and pregnancy-induced hypertension. Acta Obstet Gynecol Scand 86 (2007): 788-792.
- Bourjeily G, Danilack VA, Bublitz MH, et al. Obstructive sleep apnea in pregnancy is associated with adverse maternal outcomes: a national cohort. Sleep Med 38 (2017): 50-57.
- Jackman KA, Zhou P, Faraco G, et al. Dichotomous effects of chronic intermittent hypoxia on focal cerebral ischemic injury. Stroke 45 (2014): 1460-1467.
- Marcus NJ, Li YL, Bird CE, et al. Chronic intermittent hypoxia augments chemoreflex control of sympathetic activity: role of the angiotensin II type 1 receptor. Respir Physiol Neurobiol 171 (2010): 36-45.
- Peng YJ, Yuan G, Khan S, et al. Regulation of hypoxia-inducible factor-α isoforms and redox state by carotid body neural activity in rats. J Physiol 592 (2014): 3841-3858.
- Li T, Chen Y, Gua C, et al. Elevated Oxidative Stress and Inflammation in Hypothalamic Paraventricular Nucleus Are Associated With Sympathetic Excitation and Hypertension in Rats Exposed to Chronic Intermittent Hypoxia. Front Physiol 9 (2018): 840.
- Mao M, Yang L, Jin Z, et al. Impact of intrauterine hypoxia on adolescent and adult cognitive function in rat offspring: sexual differences and the effects of spermidine intervention. Acta Pharmacol Sin 42 (2021): 361-369.
- Fan JM, Wang X, Hao K, et al. Upregulation of PVN CRHR1 by gestational intermittent hypoxia selectively triggers a male-specific anxiogenic effect in rat offspring. Horm Behav 63 (2013): 25-31.
- Johnson SM, Randhawa KS, Epstein JJ, et al. Gestational intermittent hypoxia increases susceptibility to neuroinflammation and alters respiratory motor control in neonatal rats. Respir Physiol Neurobiol 256 (2018):128-142.
- Badran M, Yassin BA, Lin DTS, et al. Gestational intermittent hypoxia induces endothelial dysfunction, reduces perivascular adiponectin and causes epigenetic changes in adult male offspring. J Physiol 597 (2019): 5349-5364.
- Svitok P, Molcan L, Stebelova K, et al. Prenatal hypoxia in rats increased blood pressure and sympathetic drive of the adult offspring. Hypertens Res 39 (2016): 501-505.
- Song R, Mishra JS, Dangudubiyyam SV, et al. Gestational Intermittent Hypoxia Induces Sex-Specific Impairment in Endothelial Mechanisms and Sex Steroid Hormone Levels in Male Rat Offspring. Reprod Sci 29 (2022): 1531-1541.
- Sokol RZ, Okuda H, Stanczyk FZ, et al. Normative reproductive indices for male and female adult Sprague-Dawley rats. Contraception 59 (1999): 203-207.
- Lim DC, Brady DC, Po P, et al. Simulating obstructive sleep apnea patients' oxygenation characteristics into a mouse model of cyclical intermittent hypoxia. Journal of Applied Physiology 118 (2015): 544-557.
- Ribeiro RFJr, Potratz FF, Pavan BM, et al. Carvedilol prevents ovariectomy-induced myocardial contractile dysfunction in female rat. PLoS One 8 (2013): e53226.
- Xiao D, Huang X, Xue Q, et al. Antenatal hypoxia induces programming of reduced arterial blood pressure response in female rat offspring: role of ovarian function. PLoS One 9 (2014): e98743.
- Brawley L, Itoh S, Torrens C, et al. Dietary protein restriction in pregnancy induces hypertension and vascular defects in rat male offspring. Pediatr Res 54 (2003): 83-90.
- Alexander BT. Placental insufficiency leads to the development of hypertension in growth-restricted offspring. Hypertension 41 (2003): 457-462.
- Ojeda NB, Grigore D, Robertson EB, et al. Estrogen protects against increased blood pressure in post-pubertal female growth-restricted offspring. Hypertension 50 (2007): 679-685.
- Xiao D, Huang X, Yang S, et al. Estrogen normalizes perinatal nicotine-induced hypertensive responses in adult female rat offspring. Hypertension 61 (2013): 1246-1254.
- Prabhushankar R, Krueger C, Manrique C. Membrane estrogen receptors: their role in blood pressure regulation and cardiovascular disease. Curr Hypertens Rep 16 (2014): 408.
- Dubey RK, Jackson EK. Cardiovascular protective effects of 17beta-estradiol metabolites. J Appl Physiol 91(2001): 1868-1883.